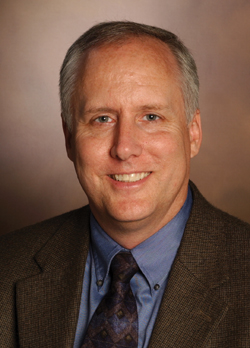
Randy Blakely, Ph.D.
Missing protein leaves mice breathless
Perhaps no neurotransmitter is as important to life as acetylcholine. Without it, your muscles don’t contract, you can’t breathe and your heart can race out of control.
This transmitter also plays an important role in the brain, influencing complex functions like learning and memory. For example, diminishing amounts of acetylcholine are linked to dementia and memory impairments in Alzheimer’s disease. Traditional drug treatments for the disease attempt to increase acetylcholine levels by inhibiting its breakdown.
Vanderbilt researchers may have a new approach for increasing acetylcholine — just recycle.
A new study by led Randy Blakely, Ph.D., suggests that the protein involved in the recycling of choline — a component used in making new acetylcholine — is as critical as the neurotransmitter itself.
In the June 1 online edition of the Proceedings of the National Academy of Sciences, Blakely and colleagues report that mice lacking this protein, the choline transporter, die shortly after birth because of their inability to make new acetylcholine.
Once acetylcholine is released from a nerve cell, it gets broken down into two chemicals — choline and acetyl-CoA. The choline then gets sucked back up into the nerve cell, where it becomes available for the synthesis of new acetylcholine.
Although the existence of a choline transporter was first postulated in the mid-1950s, it took more than 40 years to identify the actual protein. In 2000, the choline transporter was cloned in the worm, C. elegans, by a research team in Japan.
“We took our lead from that (study) and reported the identification and activity of the mouse and human choline transporter genes in 2001 and 2002,” Blakely said.
Last year, Blakely published a study documenting a surprising location of the choline transporter tucked away inside nerve cells, and how it is called into action when more acetylcholine is needed.
What they couldn’t determine at the time was just how critical this transporter was.
To investigate the importance of the choline transporter, Blakely and colleagues created mice that had only half of the normal amount of the transporter (heterozygotes) and mice that lacked the choline transporter entirely (knockouts).
What Blakely’s team observed in the knockouts illustrated the vital importance of this protein.
“Until the time they’re born, the embryos look normal, but as soon as they are born — as soon as they try to take a breath or move a muscle — the neurons that use acetylcholine start to fire and quickly run out (of acetylcholine). They become paralyzed. They can’t breath and that leads to their demise in about 30 minutes to an hour after birth,” Blakely described.
Electrophysiological studies on muscle tissues from those mice revealed the cause of their death. And it was just what Blakely had suspected.
“The knockout animals are unable to maintain stores (of acetylcholine) in the nerve terminal sufficient to continue the communication between the nerve and the muscle. That is exactly what many suspected, but hadn’t proven, the transporter’s role would be — it is required to replenish acetylcholine stores.”
The researchers tried to rescue these mice by feeding the mother extra choline during her pregnancy and by injecting drugs that prevent the breakdown of acetylcholine directly into the newborn pups. Neither of these treatments saved the knockouts from their fates. It seemed that lacking the choline transporter was a death sentence.
However, the mice that had half the amount of transporter (heterozygotes) appeared normal in almost every respect. These animals had normal choline uptake in their nerve terminals, despite having only half the normal amount of transporter protein — a surprising finding, Blakely said.
So how do these animals get by with just half the normal amount of transporter? Blakely’s recent research suggests that something is making the remaining transporter work harder, like a gas pedal being pushed down.
Blakely hopes to soon find the mechanism the transporter uses to do this. This information might indicate new strategies to treat diseases where acetylcholine production is impaired, as in Alzheimer’s disease.
Recently, scientists have identified a coding variant in the human version of the gene that diminishes the transporter’s activity by about 50 percent. This gene variant is so common that more than ten million people in the United States may carry it and a fair number carry two copies.
“What we have now is an animal model for compromised function of the acetylcholine system that might provide an important link to those individuals,” Blakely said. “Perhaps those carrying the mutation look absolutely normal but could run out of acetylcholine if stressed, say for example by the normal process of aging. Or maybe they will prove to be at risk for heart attacks.”
Blakely is currently collaborating with Stephen Lee, M.D., Ph.D., Jonathan Haines, Ph.D., and Richard Shelton, M.D., to determine whether people with disorders related to impaired cholinergic function (e.g., Alzheimer’s disease, and mood and cognitive disorders) have an increased incidence of this gene variant.
“Based on our initial mouse studies, the impact of having half the normal amount of transporter function would not be immediately obvious,” Blakely said.
“There would be no way to tell by looking grossly at a person’s ability to walk, talk or move, but when the system is challenged, say by another insult — a drug or just growing old — their cholinergic neurons may fail to respond adequately,” Blakely said.
“We think these animals will reveal other surprises regarding the choline recycling process.” Surprises, Blakely said, “that may lead to improved medications.”
Blakely is working with Jeff Conn, Ph.D., and David Weaver, Ph.D., to develop high-throughput screens to identify small molecules that could increase choline recycling and, ultimately, enhance brain function.
“After almost 50 years of suspecting this process could be vital, we now know it is,” Blakely said. “And we want to make the most of it.”
Co-authors on the study included Shawn M. Ferguson, Ph.D., Mihaela Bazalakova, Valentina Savchenko, Ph.D., and Jane Wright of the department of Pharmacology, and Juan Carlos Tapia, Ph.D., of Washington University. The research was supported by grants from the National Institute of Mental Health and predoctoral fellowships to Ferguson and Bazalakova from the Vanderbilt Brain Institute and the Medical Scientist Training Program, respectively.
NIH grants MERIT award to Blakely
by Melissa Marino
Randy Blakely, Ph.D., director of the Center for Molecular Neuroscience, has been awarded a MERIT (Method to Extend Research In Time) Award from the National Institute of Mental Health.
MERIT awards honor researchers who have shown superior competence and productivity over their research careers. The award provides up to 10 years of funding support, relieving some of the administrative burden placed on investigators by the grant renewal process. The NIMH confers only three or four of these prestigious awards annually.
“Of course I am thrilled, particularly because the award adds a level of security to one of our newer research efforts, research I believe can make an important contribution to our understanding of mental illness and neurodegenerative disorders,” Blakely said.
“I’m very pleased to hear that Randy has received the MERIT award. He’s very deserving of this recognition,” said Jeffrey R. Balser, M.D., Ph.D., associate vice chancellor for Research.
“Time freed up by MERIT awards allows investigators to focus on research rather than focusing on receiving funding for that research. Randy’s MERIT award will allow further scientific contribution to — and recognition of — the growing neuroscience research enterprise at Vanderbilt,” Balser said.
The award is based in part on Blakely’s research on the cholinergic system, a major neurotransmitter system of the brain and peripheral nervous system.
Acetylcholine, the first neurotransmitter ever identified, is also one of the most vital, controlling processes as complex as learning and memory and as basic as movement and heartbeat.
Blakely’s lab cloned the mouse and human genes that code for the choline transporter, which regulates the “recycling” of choline, an essential ingredient for the making of acetylcholine.
Blakely’s laboratory has a long-standing interest in transporter proteins, including the initial identification of the serotonin transporter, the brain’s major target for antidepressants like Prozac.
In his studies of the choline transporter, he has recently created genetically engineered mice that lack this transporter entirely (knockouts) and mice that lack one copy of the gene (heterozygotes). Using these mice, Blakely has demonstrated the critical importance of this protein.
The $3.5 million grant will support Blakely’s continued efforts to determine how the choline transporter is regulated, and whether altered choline transporter expression contributes to certain human diseases.
“We believe that these heterozygous animals are a model for some forms of cholinergic deficiency that may occur in disorders like Alzheimer’s disease, myasthenias, and cardiovascular diseases,” Blakely said. Understanding how the transporter is regulated might suggest new targets for treating such disorders.
Blakely is the Allan D. Bass Professor of Pharmacology and a Kennedy Center Investigator. Balser is the James Tayloe Gwathmey Professor of Anesthesiology and Pharmacology.
Other VUMC faculty members currently funded with MERIT awards are:
•Albert H. Beth, Ph.D., Molecular Physiology and Biophysics
•Paul E. Bock, Ph.D., Pathology and Medicine
•Alan D. Cherrington, Ph.D., Molecular Physiology and Biophysics;
•S.K. Dey, Ph.D., Pharmacology and Cell and Developmental Biology
•Raymond N. DuBois, M.D., Ph.D., Gastroenterology
•Daryl K. Granner, M.D., Biomedical Science
•Billy G. Hudson, Ph.D., Medicine
•Tadashi Inagami, D.Sc., Ph.D., Biochemistry
•L. Jackson Roberts II, M.D., Pharmacology and Medicine
•Elaine Sanders-Bush, Ph.D., Pharmacology and Psychiatry,
•Conrad Wagner, Ph.D., Biochemistry
•Michael R. Waterman, Ph.D., Biochemistry