If an unfortunate run-in with a predator leaves a salamander without a limb or its tail, in short order, the crafty amphibian can grow a perfect replica to replace the lost appendage.
The tiny flatworms, called planaria, can do one better—if cleaved in twain, they can regenerate half of their body, resulting in two fully functional and independent worms.
Nature has provided these creatures with astounding regenerative powers. Unfortunately, humans didn’t get so lucky.
Our regenerative capacity pales in comparison to the superhero-like abilities of these “lower” animals, limited to only a few tissues.
But researchers are now making progress using stem cells to repair one of the most frequently damaged human organs—the heart.
Heart attack, or acute myocardial infarction, inflicts permanent heart damage on approximately 600,000 Americans each year.
During a heart attack, blood flow to the heart is interrupted, starving the tissue of oxygen (ischemia) and damaging or killing the heart muscle cells, the cardiomyocytes. This damaged or dying tissue (the infarction) fails to properly contract and pump blood, leaving heart attack survivors with lasting cardiac failure.
Currently, there is no way to replace or renew damaged heart tissue, but recent progress in regenerative medicine using stem cell therapy is showing promise in healing these “broken” hearts.
“In regenerative medicine, we try to restore some of this lost tissue in order to save lives as well as improve the quality of life,” says Antonis Hatzopoulos, Ph.D., associate professor of Medicine and Cell & Developmental Biology at Vanderbilt University Medical Center.
Only during early embryogenesis does our innate regenerative power rival that of worms and salamanders. As development progresses, the developmental trajectories of embryonic cells become more and more restricted, limiting the regenerative abilities of most human tissues. By adulthood, only a few tissues—skin, blood and the inner lining of the gut—regenerate easily, while in most tissues, regeneration is probably very restricted or nonexistent.
Regenerative capacity is conferred by either resident populations of stem cells that can give rise to new tissue cells when needed (for example, in skin and blood), adult cells that retain the ability to divide and grow (as in the liver), or a combination of the two.
“When it comes to cardiac regeneration, it looks like the heart has none of these mechanisms,” says Hatzopoulos, who has studied the regenerative capacity of the vasculature for more than a decade.
While recent studies have indicated that the heart may have a very small reserve of stem cells, they have yet to be identified and isolated, he says, so “scientists have turned to other sources of stem cells to see if they would be able to repair damaged heart tissue.”
Bone marrow is an easily accessible and plentiful source of stem cells. The bone marrow produces several different types of stem cells including the hematopoietic stem cells, which produce the circulating blood cells; mesenchymal stem cells, which can differentiate into a variety of cell types; and endothelial progenitor cells, which repair damaged blood vessel walls.
Excitement began to build in the late 1990s, when several laboratories independently reported that circulating stem cells derived from bone marrow could differentiate into many different types of tissue cells, including the endothelial cells that line blood vessels.
Mounting evidence
Recent studies in animals have buoyed the promise of stem cell therapy for heart repair.
In a 2001 study, researchers treated mice with their own bone marrow-derived stem cells following an experimentally induced heart attack. Injection of these cells into the damaged heart tissue improved survival and led to the formation of new cardiomyocytes, vascular endothelium and smooth muscle cells, the investigators found.
“There was also clinical evidence,” says Hatzopoulos, noting earlier clinical observations of tissue regeneration in people who had previously received bone marrow transplants.
“If you look at a biopsy, you find that some of the cells that are part of the new tissue are coming from the donor (bone marrow)… indicating that there is a low level of wear and tear and replacement, and that bone marrow stem cells seem to be part of it.”
The mounting evidence triggered a number of clinical trials outside the United States—even while basic scientists were still conducting studies in animal models.
The clinical trials, which have used bone marrow-derived stem cells injected five to eight days after heart attack, have demonstrated that such therapies are safe and may offer a “moderate” improvement in heart function.
Among patients who received the cells, researchers observed an increase in the left ventricular ejection fraction (a measure of how efficiently the heart pumps blood), a reduction in the size of the infarct (indicating that the damaged heart muscle was being repaired), and an improved ability to exercise.
With these promising results, the National Heart, Lung, and Blood Institute in 2006 established a network of clinical centers to investigate this heart repair strategy in a more systematic way. Vanderbilt University Medical Center was among the network’s five founding centers—along with the Texas Heart Institute, the University of Florida, the Cleveland Clinic and the University of Minnesota.
Vanderbilt also recently enrolled its first subjects into a privately-funded study (see “Stem cell pioneer”).
Despite the promising results from the clinical trials completed to date, the clinical benefit seems modest, suggesting that the therapy has much room for improvement. So researchers are heading back to the bench.
Says Hatzopoulos: “I think the key questions in the field are: which is the best cell type, how do we get the cells to go to the desired site efficiently, how can we enhance their survival in a hostile environment, and how do we direct them into becoming the tissue cells we want?”
While bone marrow is an easily accessible and abundant source of cells, the majority of bone marrow stem cells are ultimately destined to become blood cells. A small percentage of these cells appear to develop into cardiomyocytes, but Hatzopoulos says they may require an extensive “re-education” to produce numbers adequate for significant heart repair.
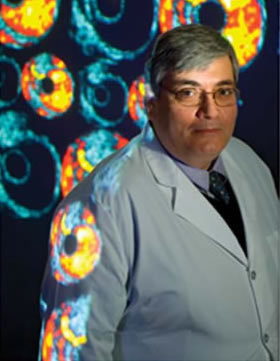
A major push in the field is to find a stem cell source that is closer to the ultimate goal of generating new heart tissue.
Mesenchymal stem cells, found in the bone marrow and other adult tissues, are known to differentiate into a variety of different cell types in the lab, including fat, cartilage, bone, muscle and nerve cells.
“Mesenchymal cells are closer to becoming heart,” says Hatzopoulos, than are other types of stem cells in the bone marrow. This places them at an advantage, but they still require some additional “push” to enhance their therapeutic efficacy.
Super stem cells
At Vanderbilt, Pampee Young, M.D., Ph.D., assistant professor of Pathology and Medicine, and her colleagues are working to identify that push. From a mouse strain that naturally exhibits a high level of regenerative capacity, Young has isolated “super” mesenchymal stem cells.
When injected into mice that have had a heart attack, they secrete factors that increase the proliferation of cardiomyocytes. Their ability to improve heart function after myocardial infarction exceeds that of injections of “normal” mesenchymal stem cells.
One factor that may play a role in their enhanced regenerative capacity is a signaling pathway known to set up the vertebrate body plan early in development, the Wnt pathway.
“We have identified a striking downregulation of the Wnt pathway in the ‘super’ mesenchymal stem cells,” Young says.
Downregulation enhanced mesenchymal stem cell proliferation, she adds, so “modulating the activity in this pathway… may be an excellent future target for cell-based therapies for myocardial injuries.”
A major obstacle is steering the cells to the desired location—the damaged heart tissue.
“In the clinical trials, in the best case scenario, no more than 3 percent to 4 percent of the cells reach the site,” says Hatzopoulos. “Most of them are lost in circulation.”
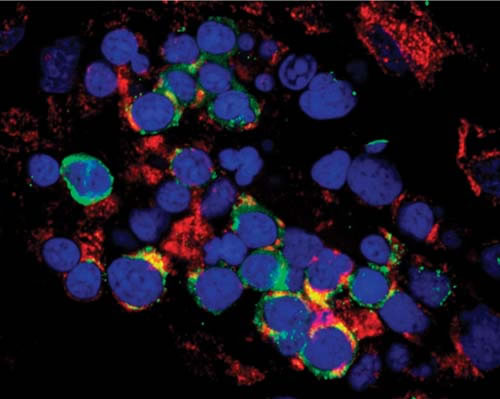
Hatzopoulos and colleagues have found that, after the ischemic event, there is an active interaction between bone marrow-derived cells and the vascular wall similar to the process seen when inflammatory cells migrate to a site of injury.
“During this process of ischemic injury, there is an upregulation of the inflammatory response,” he says. “Signals go out and mobilize a large number of cells from bone marrow—including monocytes, lymphocytes—but among them, there are cells that can participate more directly in tissue regeneration.”
The vascular wall also becomes active, or “sticky,” and captures these cells.
“The problem is that this upregulation of the ‘sticky’ vascular wall is a transient event—it happens within the first couple of days after injury and dies off,” Hatzopoulos continues.
It’s impractical to inject the cells that early “because they will be coming into a very hostile environment, so their survival is going to be very limited.” But if they’re injected a week later or a month later, “this area is not going to capture cells.”
One of the challenges, then, “is to find ways to make that homing efficient at the time you want to deliver the cells.”
The biggest hurdle of all may be inducing stem cells to become heart cells instead of blood cells. This will require a deeper knowledge of the cellular factors involved in differentiation.
Hatzopoulos and his colleagues have isolated endothelial progenitor cells from the mouse embryos and have identified about 100 proteins produced by these cells that may have “cardiogenic” properties. They are now examining these proteins in animal models to whittle down the number of factors required for the development of cardiomyocytes from stem cells.
Studying these embryonic stem cells gives researchers a “blueprint” for the differentiation of cardiomyocytes, which they can then apply to other types of stem cells to help guide their differentiation down the desired path.
“We are trying to find factors that will push these cells towards the cardiac lineage in a more effective way,” said Hatzopoulos. “The practical application…would be that if we put these factors together with stem cells and transplant them in the heart, that they will increase the yield of stem cells that become heart cells.”
If researchers are able to refine this therapy, making it more efficient and effective, stem cell therapy for heart repair could mark a new chapter in regenerative medicine.
“Many diseases—everything from heart disease to bone diseases—are so complicated, that we can’t use genes or drugs (to fix them). We have to have the cell as the building unit. The challenge is to find the right cell, and to make it do what you want it to do,” Hatzopoulos says.
“Cells are the therapeutic unit of the future,” he predicts. “We have only seen the beginning of it.”