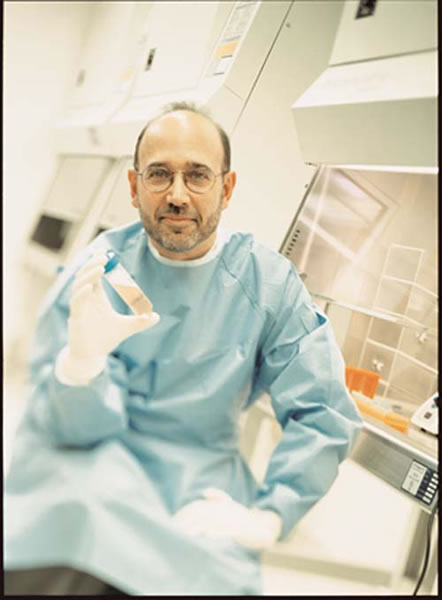
Photo by Dean Dixon
It has only nine genes, encoding just 15 proteins. Yet HIV—the human immunodeficiency virus—is a master of microevolution. So far it has evaded every attempt to subdue it.
Combinations of drugs that block key viral enzymes can reduce the “viral load” in the bloodstream to near-undetectable levels, allowing patients to live healthier, more productive, and longer lives. Yet no medical intervention has been able to flush HIV from the body or—through a vaccine—prevent infection from occurring in the first place.
“It’s highly complex, even though it’s not a living organism,” says Richard D’Aquila, M.D., who directs a new federally funded AIDS Research Center operated jointly by Vanderbilt University Medical Center and Meharry Medical College in Nashville. “It lends new meaning to the word parasite.”
HIV is not “alive” in the sense that carries the instructions necessary to reproduce. Like other viruses, HIV must hijack the reproductive machinery of the cells it infects to make copies of itself. HIV is “sneakier” than many of its viral cousins, however. Through rapid adaptation to its changing environment, the virus rapidly mutates so that some of its progeny can escape the antibodies and anti-viral drugs that otherwise would neutralize it.
Another reason HIV is so hard to stop is that it attacks “helper” T lymphocytes, also called CD4 T cells, a type of white blood cell that “orchestrates” the body’s immune response to microbial invaders. CD4 refers to a cell surface receptor to which HIV binds.
Like generals barking out orders to their troops, helper T cells send out chemical signals that “activate” the two major arms of the immune system. In one arm, B cells produce antibodies that can neutralize free-floating microbes and thus prevent them from infecting cells. In the other, cytotoxic T cells secrete a variety of toxic substances to kill cells that have already been infected.
Transmitted across mucosal barriers through sexual contact and by direct blood-to-blood contact, HIV relies primarily on a “Trojan horse” approach to reach its target host cell, the helper T cell.
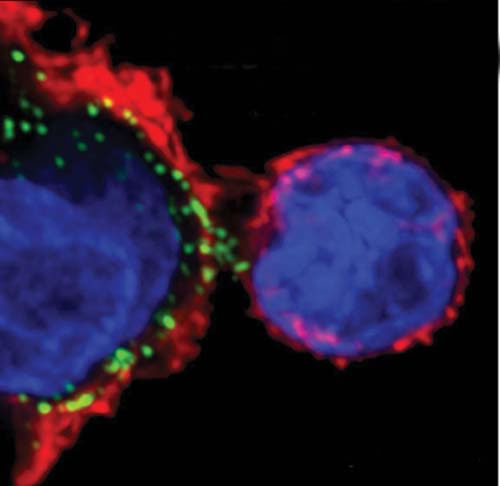
The Trojan horses are the scavenger cells, including macrophages and dendritic cells, which patrol the mucosal borders. These “border guards” normally ingest and break down viruses or bacteria, and present peptide pieces of the foreign invaders on their cell surfaces to the helper T cells. When these viral antigens dock to cell surface molecules including the CD4 receptor, helper T cells begin proliferating and activate the immune response.
Somehow HIV escapes digestion by the scavenger cells and is presented intact—and thoroughly infectious—to the helper T cell. It hooks, and fuses with, the cell membrane and pulls itself inside. Then, like others in its family of “retroviruses,” it hijacks the cell’s reproductive machinery to make new copies of itself. (See “Secrets of a Deadly Virus”).
HIV replicates faster in helper T cells that are proliferating. “The virus is so in tune with how our immune system works that it’s evolved to thrive in the exact circumstances that the immune system uses to try to beat it back,” says D’Aquila.
Eventually, and it may take years, the population of helper T cells declines—some are killed by the virus; others by mechanisms that are not well understood. When the generals are taken out of the action, immune responses gradually become uncoordinated and ineffective even against weak invaders, called “opportunistic” infections, that otherwise would not gain a foothold in the body. The result: acquired immune deficiency syndrome (AIDS).
Mutating to survive
HIV is not without its vulnerabilities. There are now more than 20 different drugs or drug combinations that can block the actions of key enzymes necessary for viral replication. More drugs are in development. Resistance is a major problem, however.
Each time HIV copies its genome, on average one error, or mutation, is introduced. Some errors are crippling: the resulting viral particle is no longer infectious. But other mutations enable the virus to hide from the immune defenses that are trying to neutralize it, or become “resistant” to the drugs that try to block it. These are the viruses that survive and thrive—because they are the fittest. “There is almost no end to the ability of the virus to change its genetic structure,” says Paul Spearman, M.D., director of Pediatric Infectious Diseases at Emory University, who is trying to understand the assembly process of the virus in order to develop an effective vaccine against it. “It’s definitely the most variable virus that we’ve ever encountered as a major pathogen.”
Not every part of HIV mutates rapidly. Some regions of a viral envelope protein called gp120 are relatively stable or “conserved,” though usually hidden from view. Gp120 exists as a trimer, three proteins bound tightly together in a sugar coat. When it binds to the CD4 receptor on the helper T cell, the trimer opens up, allowing further binding to a second “co-receptor” on the cell surface, and exposing the conserved region of the protein. Virus and cell membranes then fuse, allowing the viral contents to be “dumped” into the cell.
It’s this more vulnerable part of gp120 that Spearman and his colleagues are targeting. They’ve begun animal tests of a “pseudo-virion,” a fake (and non-infectious) viral particle, consisting of the gp120 trimer attached to the CD4 receptor in such a way that the normally hidden, genetically conserved and less mutable region of the viral receptor is exposed. The hope is that this potential vaccine will generate antibodies that recognize and attach to this viral “Achilles tendon,” thereby neutralizing the virus and preventing it from entering its target cell.
Other researchers are exploring ways to boost the effectiveness of cytotoxic T cells. Although HIV does not attack cytotoxic T cells (they bear another type of receptor, called CD8), their ability to rid the body of infected cells declines as the directions from helper T cells weaken.
Some people infected with HIV seem to be able to control the virus for long periods of time—even without drug therapy. “These are people who for whatever reason managed to get the upper hand very early,” says Spyros Kalams, M.D., director of the HIV Vaccine Clinical Research Site at Vanderbilt, whose worked helped define the critical interaction between helper and cytotoxic T cells in defending against HIV.
“I don’t know why, but everything lined up the right way, and the virus was suppressed to low levels early, before it could do much damage,” Kalams says. “We think that helper responses were preserved and they have great (cytotoxic) T cell responses and they’re maintaining control.”
Thanks to fluorescence-activated cell sorting, Kalams and his colleagues can separate—and study—distinct populations of T cells based on their tendency to proliferate and the fluorescent labels that have been attached to them. “What sort of receptors do they have? How well do they bind (to infected cells)? I’m trying to find the characteristics of each of these cells to see which ones might be the most effective at suppressing HIV replication,” he says.
Recognition of the importance of cellular immunity has led to a new avenue of vaccine development—boosting cytotoxic T cell responses. In vaccine studies in animals, these responses do not prevent HIV from infecting cells, but they can slow down the course of the disease. “It is likely that a successful HIV vaccine will have to elicit both antibody and cellular immune responses,” Kalams says.
Toward that end, Merck & Co., one of the world’s leading vaccine manufacturers, is testing a circular bit of DNA called a “plasmid” that contains a viral gene. The plasmids are injected into muscle. Infected muscle cells are engulfed by the body’s border guards, which transcribe and translate the genetic material into pieces of viral protein. The hope is that these antigens will activate cytotoxic T cells to attack HIV-infected cells.
A multi-pronged attack
At the National Institutes of Health, the Vaccine Research Center is developing a two-stage vaccine: a plasmid expressing modified genes that cover about 80 percent of the antigen content of HIV, followed by a booster consisting of the same genes carried in a harmless adenovirus. The genes are carried into cells by the adenovirus where they produce the simulated viral proteins that are processed and presented on their surfaces.
In early studies in animals and uninfected humans, this approach has triggered significant increases in both cytotoxic T cells and helper T cells, says Barney Graham, M.D., chief of the Viral Pathogenesis Laboratory and Clinical Trials Core at the NIH Vaccine Research Center.
Combining a variety of HIV antigens into one vaccine may help prevent the virus from sneaking around the body’s defenses by changing its highly mutable envelope protein. And it may provide protection against different “clades” or species of the virus. Graham hopes the vaccine may be ready for phase III clinical trials, which will measure its ability to reduce or prevent infection in high-risk individuals, by 2006.
HIV’s resilience is due not only to its astounding ability to adapt to its environment. The virus also can hide from the immune system and drug treatment in the DNA of non-dividing T cells. When the cells are activated, years or even decades later, the previously latent virus re-emerges.
Another problem is that cellular proteins called transporters can prevent the drugs from even getting to the virus in the first place. Transporters are designed to keep cells clean, by pumping out toxic materials—but they also can eject anti-retroviral drugs.
Genetic differences in individuals also seem to play a role in the effectiveness of drug therapy, and the severity of side effects.
David Haas, M.D., principal investigator of the Vanderbilt AIDS Clinical Trials Center, and his colleagues have found that African-Americans are six times more likely than Caucasians to have a polymorphism, or genetic variation, that limits the ability of a cellular enzyme to break down a common AIDS drug. The result: higher levels of the drug and a greater frequency of neurocognitive side effects, including mental confusion.
All this makes therapy extremely complicated. Doctors must isolate the virus from their patients, determine its genetic sequence in the laboratory, and pick the drugs that are most likely to inhibit that particular strain of HIV. “It’s individualized therapy,” says D’Aquila, who helped pioneer HIV sequencing technology and resistance testing. “It’s in constant flux. Every couple of months there’s some new earth-shaking development.”
The good news is that there are a lot of tantalizing leads to follow: natural factors and other compounds that can block HIV inside the cell; advanced technologies that enable scientists to “see” what’s going on at the cellular and molecular levels; and new ways of boosting immune responses.
“I have great optimism that with some of the brightest minds of our generation focused on this problem, that we will have an effective AIDS vaccine,” Haas says. “I envision one day in the distant future when we have a single pill that treats HIV in almost all people. But … the only way we’re going to get to that is with continued, vigorous support of research.”