Editor’s Note: This story, first published in 2004, has been updated.
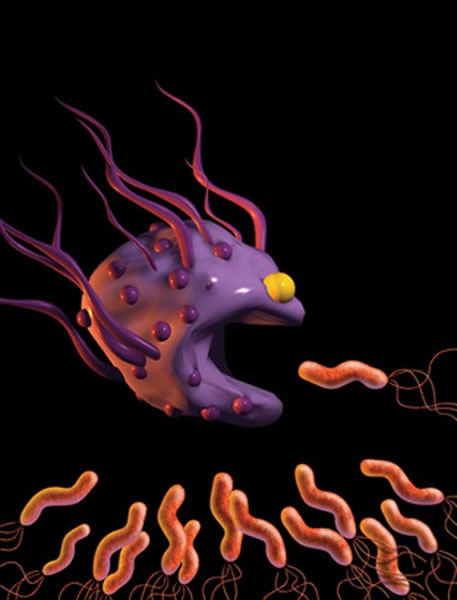
In a world brimming with disease-causing germs, inflammation is our body’s best defense against dangerous microbes. However, when it goes awry, it can also be our worst enemy.
Humans have been battling inflammation since the dawn of time. Yesterday’s willow tree bark is today’s plethora of pills and tablets. According to IMS Health, a pharmaceutical information and consulting company, more than $17 billion dollars are spent annually in the United States on prescription anti-inflammatory drugs.
The quest for better medicine continues today. Dozens of compounds are moving through the drug-development pipeline to address a growing list of inflammatory conditions.
Meanwhile, scientists are deciphering the interplay of cells, chemical messengers and genes that makes up the language of inflammation. Along with this understanding comes an increased capability to intervene—to treat and ultimately to prevent the disabling, potentially life-threatening and costly consequences of chronic inflammation.
The language of inflammation
Inflammation begins when white blood cells—mast cells, neutrophils, macrophages—residing in tissue respond to the site of an injury or infection. They produce waves of chemicals—called inflammatory “mediators”—that can kill germs and sound the alarm for other populations of inflammatory cells.
Unfortunately, these mediators also “can put tissue on ‘fire,’ and cause ‘collateral damage,’” says Jacek Hawiger M.D., Ph.D., who is leading the search for inflammation-related genes at Vanderbilt University Medical Center.
Inflammation is a complex, multiple signaling phenomenon, but it can be broken down into three basic groups of mediators: reactive oxygen species (ROS) such as hydrogen peroxide and “free radicals” (atoms with unpaired electrons); eicosanoids including the prostaglandins and leukotrienes; and protein messengers called cytokines.
The production of free radicals by neutrophils and macrophages is called oxidative or oxidant stress, and it is a critical part of infection control.
Oxidative stress goes hand in hand with inflammation. The first response is the neutrophil or macrophage engulfing a bacterium. Then, as a consequence, is the oxidative burst that leads to the generation of hydrogen peroxide, superoxide and other free radicals that acutely damage the invading organism.
Free radicals are important in killing bacteria, but, in doing so, they can damage surrounding tissue. Oxidative damage has been linked to the development of Alzheimer’s disease, certain forms of cancer, and other inflammatory diseases, which suggests that antioxidants might be useful in preventing or treating such disorders.
Free radicals don’t last very long in tissues, making it difficult to measure their impact directly. However, their damage can be assessed indirectly. At Vanderbilt, the late Jason Morrow, M.D., and his colleague, L. Jackson Roberts, M.D., identified and characterized a unique class of oxidatively-damaged fats, called isoprostanes. Circulating levels of isoprostanes provide an accurate and non-invasive index of oxidative stress in humans.
Morrow and colleagues at Oregon State University also found that the extreme exercise involved in an ultra-marathon (a 32-mile run) caused increased production of isoprostanes and inflammatory cytokines. Runners who took high doses of antioxidant vitamins had significantly lower levels of isoprostanes in their blood compared to runners who took a placebo, but the vitamins had no effect on measures of inflammation.
“We de-linked the protein (cytokine) component of inflammation from the oxidative stress component,” Morrow said in 2004. “This says that inflammation is a multi-factor pathway and not all of those pathways are regulated in the same way—if we block one pathway (such as oxidative stress) we may still have another pathway that can be damaging.”
One of the other potentially destructive arms of inflammation involves lipid-derived molecules called eicosanoids. These molecules, which include the prostaglandins and leukotrienes, are produced by nearly every cell in the body. They act as local hormones, regulating many biological functions including smooth muscle contraction.
Prostaglandins are also released by damaged cells and by macrophages, and they contribute to the cardinal signs of inflammation. They stimulate pain receptors in the damaged tissue, promote vasodilation (heat and redness), and increase capillary permeability, leading to the accumulation of fluid in tissue (swelling).
Prostaglandins are made from arachidonic acid by the cyclooxygenase (COX) enzymes, which come in two forms. In the early 1990s, researchers determined that prostaglandin production by one of the enzymes, called COX-1, protects the stomach lining, whereas activation of a related enzyme, COX-2, in other tissues can lead to inflammation, pain and tumor growth.
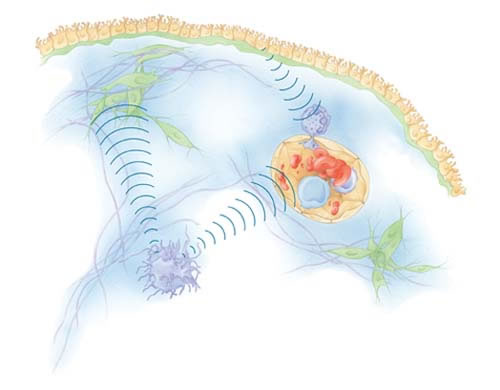
The finding led to the development and the marketing of the blockbuster arthritis drugs Celebrex and Vioxx, which specifically inhibit the COX-2 enzyme without affecting the activity of COX-1. Thus, they were designed to relieve pain and inflammation without causing stomach upset and gastrointestinal bleeding, a problem with aspirin and other non-steroidal, anti-inflammatory drugs (NSAIDs) that block both enzymes.
The third arm of inflammatory mediators includes the cytokines, a large family of messenger proteins that—among other things—are involved in reproduction, the development of the embryo, maturation of blood cells and the immune response.
Cytokine production also is an essential part of the inflammatory response to infection or injury. Produced by a variety of cells in response to injury or infection, they communicate messages that help coordinate the processes of healing and fighting pathogenic invaders.
Cytokines involved in the inflammatory response include the interferon and interleukin families and tumor necrosis factor-alpha (TNF-alpha). Interferons and interleukins play a variety of roles; some of them “fuel the fire” of inflammation, while others dampen it.
Various treatments are being developed to either block pro-inflammatory members of the interleukin family or enhance the anti-inflammatory activities of others.
TNF-alpha, named for its ability to kill tumor cells, is thought to be at the center of inflammatory signaling. It can help recruit white blood cells to the site of inflammation, thereby boosting production of perhaps dozens of other cytokines.
Within the last few years, several drugs that shut off TNF-alpha signaling have come to market for the treatment of rheumatoid arthritis and other chronic inflammatory disorders such as Crohn’s disease. Since TNF-alpha signaling appears to be altered in a number of other inflammatory conditions, including cancer, multiple sclerosis and diabetes, the impact of these drugs may be far-reaching.
Stopping the cytokine storm
Cytokines are not the only chemical messengers involved in inflammation. Chemokines and cell adhesion molecules help attract and direct the “first responders” to the source of injury or infection, and to bring in the reinforcements once the inflammatory response has begun.
These factors aren’t always around, however. “They have to be produced on demand—just in time when you have inflammation,” says Hawiger, the Oswald T. Avery Distinguished Professor of Microbiology and Immunology at Vanderbilt and chair of the department.
To provide inflammatory signals only “as needed,” the genes that express them must be switched on and off. This realization has led Hawiger and his colleagues to the nucleus where, ultimately, inflammation begins.
The gene switch is operated by “stress-responsive transcription factors,” proteins that—upon activation by pro-inflammatory stimuli such as oxidative stress—slip into the nucleus and turn on the genes for inflammatory cytokines, chemokines and the like.
Hawiger’s group has developed a peptide or protein fragment called cSN50 that can keep the transcription factors out of the nucleus. This brings production of inflammatory messengers—what is commonly called the “cytokine storm”—to a screeching halt.
Recently they tested cSN50 in mice exposed to a bacterial toxin that can cause a life-threatening systemic inflammatory response called toxic shock. By shutting down cytokine and chemokine production, the peptide dramatically reduced liver damage and mortality, they reported in April.
The genomic approach may have broad implications, since excessive cytokine and chemokine production is a central theme in every inflammatory disease. About 250 genes that mediate inflammation have been identified so far. That’s just the tip of the iceberg, Hawiger says.
In 2001, a multidisciplinary group of Vanderbilt researchers led by Hawiger was formed to find genes that mediate inflammation, with the ultimate goal of identifying new targets for anti-inflammatory drugs. Funded by a $10 million grant from the National Heart, Lung and Blood Institute, the “Functional Genomics of Inflammation” program so far has developed a number of tools—including gene arrays, bioinformatics techniques and “knockout” mice—to aid in the search for the “inflamed gene.”
“We are currently pursuing between eight and 12 genes,” Hawiger says. The scientists plan to characterize the proteins expressed by these genes using proteomics techniques to determine their precise roles in inflammation.
“This … will help us to solidify a new paradigm of inflammation based on its ‘control center’ in the cell’s nucleus,” Hawiger says. “Thus, we will move closer to the development of a new generation of more effective and, hopefully, safe anti-inflammatory drugs tailored to tackle the key gene players in inflammatory cells.”