Editor’s Note: This story, originally published in 2005, has been updated.
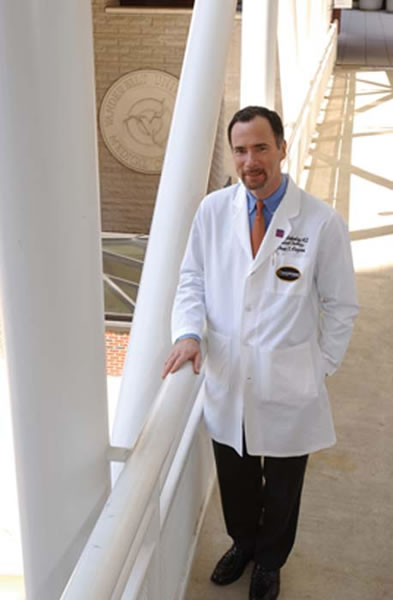
An informal search of medical news Web sites on any given day will typically return dozens of reports on the discovery of a new cancer-related gene, yet between 2000 and 2005 only five new agents were approved for the treatment of cancer.
With so many new molecular and genetic targets being identified, why is the pace of cancer drug development so slow?
Leading researchers believe current design and analysis of clinical trials may contribute.
In a 2003 review in Nature Reviews Cancer, Vanderbilt cancer researchers Mace Rothenberg, M.D., David Carbone, M.D., Ph.D., and David Johnson, M.D., identified factors that may be prematurely sending some potentially useful cancer drugs to an early grave.
From a large volume of pre-clinical studies, compounds that inhibited angiogenesis, the formation of new blood vessels, appeared to be the “dawn of a new era” in cancer therapy, describes Rothenberg, professor of Medicine and Ingram Professor of Cancer Research.
Laboratory studies suggested that angiogenesis inhibitors alone could be used to cure cancer. Because blood vessel formation was one of the decisive factors in tumor growth, researchers thought that inhibiting this process would kill tumors by robbing them of their blood supply. Also, angiogenesis inhibitors were thought to be much less toxic than standard chemotherapeutics.
When the first angiogenesis inhibitor trials began, an ensuing hysteria—in the form of enrollment “lotteries” and patients traveling cross-country to participate—followed. The frenzied expectation surrounding these drugs soon diminished as the evidence began coming in from the clinical trials. When used alone, the first generation of angiogenesis inhibitors were not clinically effective.
These “failures” forced scientists to rethink their strategy. The tide shifted to testing angiogenesis inhibitors as an adjunct to conventional cytotoxic therapies and to developing better angiogenesis inhibitors. This strategy proved much more effective, leading to the approval of the first angiogenesis inhibitor, Avastin, in 2004.
“Animal/preclinical models have been very useful in helping us explore the biology of cancer, but we have very few useful models that have been developed that recapitulate the complexity of human cancers in the clinical setting,” Rothenberg explains.
“I think the mistake has been in assuming that these results will accurately predict clinical effect. They do not.”
In the 1990s, researchers optimistically predicted that targeted therapies—drugs designed to act only on a single cellular component—would be more effective and would eventually replace conventional cytotoxic therapies. Since targeted therapies would only affect cells that harbored the specific target, the drugs also would be safer and cause less damage to normal tissues.
This hypothesis was based largely on trials of Herceptin, the first growth factor-targeted monoclonal antibody approved to treat breast cancer. It prevents epidermal growth factor (EGF) from binding to a receptor known as Her2.
“At a very crucial time in its development, it was recognized that only those tumors that had significantly high levels of expression of the receptor Her2 would likely respond,” Rothenberg says. Because of this, the clinical trials were limited to patients whose tumors overexpressed Her2, and quickly showed that it was a beneficial agent to incorporate into therapy.
However, when the same strategy was tried with another receptor that binds EGF, Her1, “Lo and behold, this didn’t carry over,” he says.
Her1-targeted drugs such as Iressa, Tarceva, and Erbitux showed a similar activity in all patients, whether their tumors expressed low, intermediate or high levels of the receptor. Even patients whose tumors didn’t express Her1 benefited from Erbitux.
Then in 2004 scientists discovered that patients were more likely to respond to Iressa or Tarceva if they had a mutation in the Her1 receptor.
“So that taught us an important lesson,” Rothenberg says. “Even though we talk about targeted therapies, different targets may have different rules of engagement. We can’t make a sweeping assumption that what is true in one is true in all.”
Underlying the unpredictability is a significant amount of crosstalk between numerous cell-signaling pathways.
“Shutting down one pathway doesn’t necessarily lead to death of the cancer,” he says. “Other regulatory pathways may be abnormally functioning in these cells. Therefore, our approach currently is to target complementary pathways, so that we can more completely shut down those growth factor signaling pathways that are driving the cancer cell.”
Rothenberg and his colleagues also are using pharmacogenomic profiling to define the biology that drives the clinical activity of drugs, both the anti-tumor activity and toxicity.
“To relate this information about drug response to genomic or proteomic profile of the tumor will give us hopefully more useful and predictive information about what drugs might be useful in what doses and in what setting,” he says.
“We are at an unprecedented time of opportunity in the field of drug development,” Rothenberg concludes. “The gap that has existed for many years—between what we understand about the science of cancer and the way we treat cancer—is narrowing.”