Editor’s Note: This story, first published in 2006, has been updated.
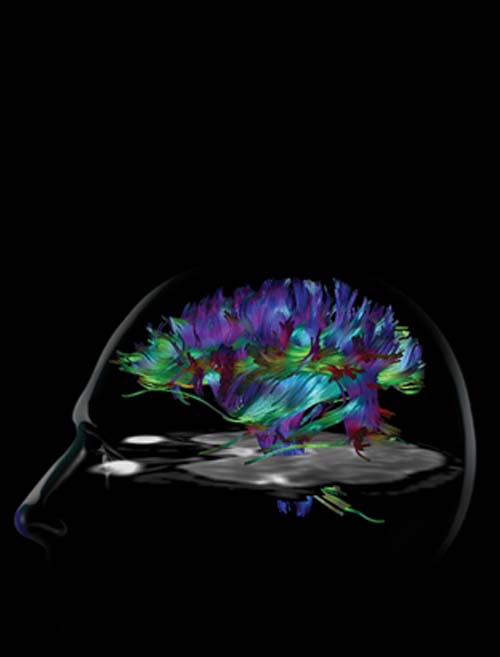
Since the discovery of the X-ray, scientists have tried to take pictures of the mind at work.
One hundred ten years later, they have never been closer.
Soon it may be possible to predict—and avert—the development of drug addiction, to individualize therapy for schizophrenia and other disorders, and to preserve and even augment brain function.
“Traditionally, imaging meant radiology—you went to the X-ray department. Imaging is now much more broadly based,” says John C. Gore, Ph.D., director of the Vanderbilt University Institute of Imaging Science.
Now, techniques like functional magnetic resonance imaging (fMRI) and positron emission tomography (PET) provide much more than a static snapshot of the brain’s form. They can illustrate the symphony of activity that underlies memory, addiction and love. They can also resolve dysfunction related to psychiatric and neurological disease and watch how drugs and educational interventions “normalize” brain activity.
“Can we measure the effect of a behavioral therapy? Can you tell whether someone is going to recover from aphasia? Those are questions that radiologists have not previously thought much about,” Gore says. “They now have the tools to do it.”
The explosion of fMRI studies in psychiatry and psychology has revealed volumes of information about individual brain regions and their principal functions.
While this may convey the rather simplistic notion that the brain is highly compartmentalized, another imaging technique based on MRI, diffusion tensor imaging (DTI), may help weave these isolated islands of brain activity back together again into a functional network.
“With fMRI, you can look at brain function—where in the brain certain information is processed,” says Adam W. Anderson, Ph.D., associate professor of Biomedical Engineering and of Radiology at Vanderbilt. “With DTI, you can look at the connection between areas of the brain that are processing that information.”
From the energy emitted by the nuclei of hydrogen atoms in the presence of a magnetic field, MRI can generate exquisitely detailed images of soft tissues, including the grayish parts of the brain that contain the neurons (called “gray matter”).
DTI, on the other hand, illuminates “white matter,” bundles of long fibers (axons) that transmit signals between different parts of the brain. In particular, the technique measures “anisotropy,” the degree to which water movement is greater along fibers than in other directions.
Anisotropy may help explain why some patients with schizophrenia experience auditory hallucinations. In 2005, Swiss researchers reported finding increased anisotropy in the brains of patients who frequently heard voices, particularly in the white-matter tracts that connected hearing and language centers.
Increased anisotropy is a measure of greater connectivity in the axon bundles. Neurons that are too strongly coupled to each other may fire off signals too readily, resulting in over-activation of parts of the brain that process external sounds and language, the researchers reported.
For patients with schizophrenia, this may explain the inability to distinguish their own, self-generated thoughts from external speech.
The Swiss report, entitled “Pathways That Make Voices,” reinforces the value of DTI in exploring other neurological and psychiatric diseases, says Anderson.
In collaboration with other Vanderbilt researchers, Anderson is using the technique to investigate known white-matter diseases such as multiple sclerosis, and to determine the effects of prenatal cocaine exposure on brain connectivity.
DTI and other MRI techniques also are proving useful to understanding how the developing brain is wired, and how conditions such as premature birth can affect cognitive development. The goal is to find ways to prevent “anatomic disturbances” from impairing “functional capacities,” according to Anderson, Gore and their colleagues at Yale.
“Neuropsychologists have spent a lot of time looking at the brain as it falls apart from injury,” adds imaging pioneer Marcus E. Raichle, M.D., professor of Radiology, Neurology and Anatomy & Neurobiology at Washington University School of Medicine in St. Louis. “But it would be equally important to watch it being put together during development.”
Right drug, right dose
Schizophrenia remains one of the most challenging brain disorders. Characterized by delusions and hallucinations, the illness disrupts cognition and emotion, profoundly affecting a person’s ability to think clearly and interact with others.
Imaging techniques, including PET and fMRI, are aiding understanding of the disease and the development of drugs to treat it.
The classic antipsychotic drugs, such as Thorazine, block receptors for the neurotransmitter dopamine, which also is centrally involved in Parkinson’s disease, addiction and other brain conditions. But while the drugs squelch the delusions and hallucinations, in high doses they also cause Parkinson’s-like symptoms, including rigidity and loss of muscle control, and they don’t improve cognitive function.
In the early 1990s, Herbert Y. Meltzer, M.D., and colleagues at Case Western Reserve University helped establish that clozapine and other second-generation “atypical” antipsychotics could effectively treat psychosis and improve cognition without Parkinsonism. This was great news, but how did the two classes of drugs produce such different effects?
This is where imaging came in.
Robert M. Kessler, M.D., and his colleagues at Vanderbilt had developed a number of radiolabeled compounds visible on PET scans that bound to a particular dopamine receptor, called D2, if it was not already occupied by an antipsychotic drug. In this way, the researchers could generate pictures of where in the brain the drugs were working.
Working with Meltzer, who currently directs the Division of Psychopharmacology at Vanderbilt, the researchers found that while the older drugs block D2 throughout the brain, the atypical class selectively binds to receptors in the cortex, the center of higher brain function, but not in areas involved in motor function.
“It’s a surprise nobody expected,” says Kessler, director of the Vanderbilt Center for Molecular Imaging. “It is the same receptor, the same protein” in both places.
The Vanderbilt researchers have also used fMRI to study the effect of drug treatment on specific cognitive functions, such as short-term working memory. By illuminating changes in blood flow and oxygenation, fMRI can create “pictures” of how the brain works under various treatment conditions.
Because individual patients respond differently to different medications, molecular imaging may one day help identify the best drug for a particular patient.
“We may find that schizophrenia is not one disease, but several different types,” Kessler says. “We may be able to determine what factors predispose one to being treated by one class of drugs as opposed to another.
“We may be able to individualize therapy, provide better dosing so that the side effects are spared and the therapeutic benefits are enhanced.”
Finding the right dose of the right drug is critical, says Ronald Baldwin, Ph.D., research associate professor of Radiology at Vanderbilt.
“A lot of people are actually overdosed,” says Baldwin, who develops novel radiotracers to probe the actions of drugs in the brain (See “How to Make an Atomic Drug”). “They are getting more drug than they need to get an effect. With radiotracer imaging, you can look at the receptor that’s binding the drug to see how much drug is occupying it.”
Mysteries of music and math
Lyric soprano Gloria Lenhoff has sung in opera houses and performance halls all over the country. Her repertoire spans more than 2,000 pieces in 30 languages, yet she can’t read music.
Lenhoff has Williams syndrome, a rare neurodevelopmental condition characterized by mild-to-moderate mental retardation, blood vessel disease and—among other surprising traits—an affinity for music.
The genetics of the disorder are well known, but a chromosomal aberration that slices out a specific a region of chromosome 7 cannot explain Lenhoff’s unique gifts.
Brain imaging may help.
Researchers at Stanford University, for example, have used fMRI to study the brains of people with Williams syndrome while they listened to snippets of Bach, Beethoven and Mozart. In 2003, they reported finding “strikingly different” patterns in the way the brains of people with Williams syndrome processed music, compared to normal controls.
When the control group listened to music, the hearing center in the brain’s temporal lobe lit up. Among people with Williams syndrome, however, brain activation was more widespread, and included the amygdala, an almond-shaped structure near the base of the brain that plays a key role in emotions.
The finding may provide clues to the comforting power of music, says Elisabeth M. Dykens, Ph.D., associate director of the Vanderbilt Kennedy Center for Research on Human Development.
Dykens, a professor of Psychology and Human Development, has studied the personality characteristics of Williams syndrome and other developmental disorders for several years.
People with Williams syndrome are prone to heightened anxiety and fear of loud noises. At the same time, they can be exceedingly social, highly verbal and friendly.
Dykens found that those who were more musically involved tended to be less anxious and fearful. The goal now is to try to link this observation to specific areas of the brain. “We hope to see how this connection plays out in the imaging studies,” she says.
Functional MRI also is proving to be a powerful educational tool.
Gore was among the first to apply fMRI to evaluate reading disabilities while at Yale University, where he directed the Nuclear Magnetic Resonance Research Center.
Children with dyslexia have difficulty recognizing and comprehending written words. Rhyming games and other drills that break down and rearrange sounds to produce different words can help them improve their reading skills.
With fMRI, “you can actually see that in the brain, in the circuits involved in reading,” Gore says. Sequential brain scans indicate that brain activity in these children actually “normalizes,” or begins to resemble activity seen in children without dyslexia.
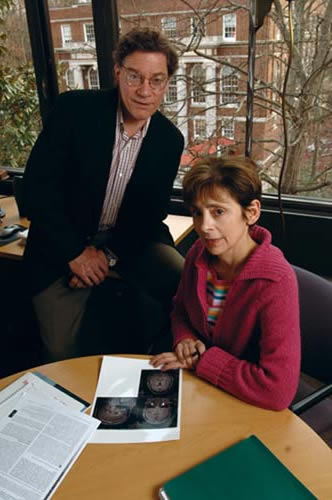
Gore, who came to Vanderbilt in 2002, is now working with investigators at the Kennedy Center to extend that work to children with math learning disabilities.
Researchers believe math disability—difficulty in solving math problems—may be a different syndrome or have a different cause than reading disabilities like dyslexia, says Lynn S. Fuchs, Ph.D., who, with her husband, Doug Fuchs, Ph.D., has helped pioneer the diagnosis and treatment of learning disorders.
The Fuchses, who share the Nicholas Hobbs Chair in Special Education at Vanderbilt, and Donald L. Compton, Ph.D., associate professor of Special Education, are using fMRI to assess how brain function changes in response to an intervention aimed at improving math skills.
“The reason to do the scanning is… to understand how the brain is changing as math improves,” Gore explains. “Is it changing in a way that makes it look more like a normal brain or is it changing in a way that compensates for some kind of structural problem that really can’t be changed with intervention?
“If you can actually train parts of the brain to do the job the most efficient way,” he adds, “then that is the best thing to do. Imaging can identify optimal strategies.”
Predicting relapse and recovery
Addiction is another challenging medical problem that is slowly revealing its secrets to brain imaging.
Through the use of PET and, more recently, fMRI, scientists are beginning to define—anatomically and biochemically—what drugs of abuse do to the brain.
“We’ve known for probably 30 years or more that using drugs like alcohol for long periods of time causes injury to the brain,” says Peter Martin, M.D., professor of Psychiatry and Pharmacology and director of the Division of Addiction Medicine in the Department of Psychiatry at Vanderbilt.
In computerized tomography (CT) scans, for example, “the brains of alcoholics seem to have shrunk compared to normal,” he says. Yet images of the brain’s structure don’t correlate well with impairments detected in neuropsychological tests. So, in the early 1990s, “we started looking at what was happening chemically in those regions of the brain that shrink.”
Martin and his colleagues used a magnetic resonance technique called MR spectroscopy to measure several brain chemicals including N-acetylaspartate (NAA), which is found primarily inside neurons (nerve cells). The researchers detected lower levels of NAA in the cerebellum in alcoholics, compared to normal controls, suggesting the presence of damaged or dying neurons.
The cerebellum, located at the base of the skull, controls muscle tone, balance and fine movement coordination. It also transmits signals to the prefrontal cortex behind the eyes, which plays key roles in working memory and judgment, as well as emotion, arousal and attention.
Levels of NAA were lowest—indicating the greatest amount of neuron damage—in the cerebella of patients who had started drinking heavily at an earlier age and who had a family history of alcoholism. These patients also relapsed earlier after a period of abstinence than did alcoholics with higher NAA levels, the researchers reported in 2002.
“It’s almost as if their brains were more sensitive to developing brain injury,” concludes Martin. “In the future, we may be able to do spectroscopy and predict which patients will do well and which ones will not.”
Imaging technologies such as fMRI not only can record damage done by drugs; they are shedding light on the processes in the brain—including reward, motivation, memory and craving—that can lead to and maintain addictive behavior.
Functional MRI measures the magnetic properties of hemoglobin, the iron-bearing oxygen transporter in red blood cells. Activation of part of the brain increases the demand for oxygen, and thus the intensity of the intensity of the magnetic signal.
Ronald L. Cowan, M.D., Ph.D., assistant professor of Radiology and Radiological Sciences at Vanderbilt, is using fMRI to study how brain activation changes over time in response to amphetamine, a powerful stimulant.
By taking a series of images, he hopes to “picture” different stages of drug-taking behavior—from the anticipation of getting the drug, through the experience of euphoria, or the “high,” and as the effect of the drug wears off.
“We have little idea what… causes a person to decide to engage in a rewarding activity, be it eating, sex, gambling or drugs,” Cowan explains. “We don’t know what parts of the brain are involved in the initiation of that activity.
“We’re hoping to find a part of the brain early on that gets activated before the experience of euphoria, that actually predicts that it is going to happen,” he says. “That might give us a target for therapy, or at least for further study.”
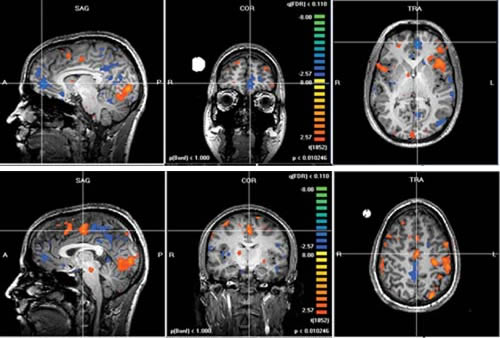