What is developmental biology? With respect to human health, why are we so excited about findings being made under this discipline’s umbrella, sometimes in model organisms as lowly as tiny worms and fruit flies?
Developmental biology encompasses studies of all organisms, plant and animal, large or small, and whether they are composed of a single cell, clusters or billions of cells. Although focusing largely on early development, embryogenesis and organ formation, in its broadest interpretation the field covers the entire life cycle, including aging and death.
In our modern molecular era, developmental biologists use any analytical technique they can lay their hands on to get a better understanding of the various building blocks and assembly instructions for life: How oocytes and eggs form, how fertilization creates the zygote, and then how embryos in each species develop the right shape with organs all correctly placed.
This remarkably high-fidelity process uses genetic programming to ensure that each organ develops the proper proportions of cell types with complex interconnections to allow physiological function over what, in humans, can be almost a century of activity.
One day we hope to hold in our hands a comprehensive, minutely detailed catalog of how thousands of molecules, in multitudinous interactions, create neurons or insulin-producing cells. This molecular blueprint is the cell’s version of the operating system of the most complicated computer ever built.
This exciting undertaking is of course daunting, even more so because the biological operating system invented by nature is very flexible and versatile. We will need to learn how it changes according to the stage of developmental process, and at a grander level, how it drives evolution. Furthermore, cells are always extremely busy communicating with each other throughout embryonic development and organogenesis, and it is really critical to connect these interactions to the nuclear activity going on in each cell.
By trying to understand how normal development occurs, we also can find out a lot about what can go wrong, with potential for future therapies for congenital disorders, autoimmunity diseases, aging and even cancer. A wide range of animals and organs, and topics, are covered in this issue of Lens.
Previous to this current age of completed genome sequences, and before “developmental control genes” had even been found, it was to some people bordering on silly to suggest that organisms all the way from nematode worms and insects, through amphibians, birds and mammals would use basically the same kinds of genes to guide embryogenesis and organogenesis.
This fundamental principle is now, however, relatively well established.
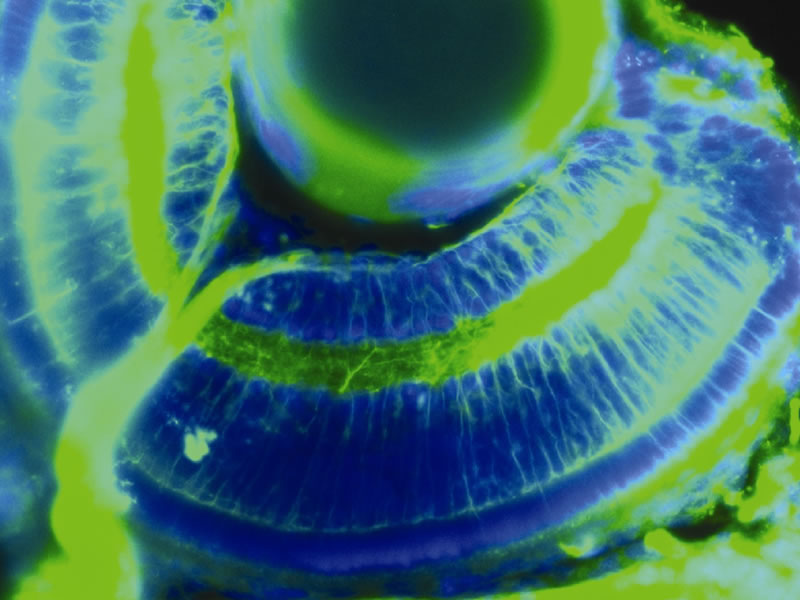
Organisms seem to become more sophisticated by developing new combinations of a basic toolkit of molecules and mechanistic subroutines that control cell formation and interactions. New versions of proteins can arise via the duplication of parts of chromosomes carrying certain developmental control genes, with the protein’s sequence and properties then diverging (mutating) slightly. In addition, the gene control sequences that dictate timing and location of protein production can be modified to produce new functions.
Elegant mechanisms
In its beginning, developmental biology was founded upon precise and rigorous descriptive work, a scholarly tradition that lingers to this day. The now-classic experimental embryology of the early 20th century was conducted by a small “club” of the well-to-do who could finance their own inquiries. These pioneering scientists used genetics or direct manipulation to find out how tissues interact to produce the different parts of the embryo.
Today’s science, while remaining grounded in precise “descriptology,” is more egalitarian, thanks largely to government support. We also have moved into a period where genetics is combined with the most modern biochemical and cell biological methods. Over the last two decades, we have gained the power to assess directly, and in the actual developing tissue, which genes (and even which exact region of their DNA) are being bound to and activated by the development-regulating proteins. I predict that we will soon have amazing multiplex renditions of such information for hundreds of proteins at the same time.
The accomplishments and contributions of developmental biology are easy to recognize in reviewing the list of Nobel laureates in medicine: from the fruit fly geneticist Thomas Morgan (1933) and amphibian embryologist Hans Spemann (1935), to Barbara McClintock, recognized for her work on mobile genetic elements in maize (1983), and Edward Lewis, Christiane Nusslein-Volhard and Eric Wieschaus (see article on E.W. in this Lens), for the genetics of fruit fly embryogenesis (1995).
These were followed by Sydney Brenner, Robert Horvitz and John Sulston (2002), honored for their studies on organ development and programmed cell death in nematode worms, and Richard Axel and Linda Buck (2004), for discovering the elegant molecular mechanisms of mammalian olfaction.
Among the most recent Nobel laureates: Andrew Fire and Craig Mello (2006), whose findings in C. elegans on gene regulation by short RNAs sparked another revolution in methods for manipulating gene activity; and Mario Capecchi, Martin Evans and Oliver Smithies (2007), recognized for their terrific work on how to engineer targeted mutations in genes using embryonic stem cells.
Discoveries by developmental biologists regarding stem cells stand among the most spellbinding. In their full incarnation, stem cells have almost unlimited proliferation capacity and long lifespan, and their “pluripotency” enables them to put out many different cell types. They have, especially as embryonic stem cells, led to much scientific, political, and ethical/moral debate.
Because we are now starting to find out how to control the differentiation of stem cells down specific paths, which could herald the large-scale production of material suitable for cell-based therapies for many kinds of human disease, developmental biologists of all ages (professors and trainees) have a responsibility to get properly informed and to teach others on these issues.
Early stem cell work involved trying hard to generate tissue culture dish models for embryonic development, and it was essentially this track that has led to our ability to manipulate the genome in stunning ways. In mice, one can now choose from a delightful smorgasbord of ways to manipulate any chosen gene. These include mutations to inactivate it or to engineer individual amino acid alterations to create models of human syndromes, or to add a fluorescent protein tag that allows us to watch the gene turning on or off in the embryo, and even to pull the cells out very selectively for analysis in vitro.
Sense of wonder
There is a justifiable current furor among scientists over the discovery that a special set of just three or four genes can impart the property of pluripotentiality to mature, differentiated cells. This property is usually associated with embryonic cell types. Defining how the necessary changes in gene activity are effected at the level of chromatin organization in the nucleus is an extremely active area of research, with implications at many levels.
Such epigenetic regulation and reprogramming is really attractive, given the prospect of being able to induce regeneration from a patient’s own cells to replace those destroyed by Alzheimer’s, muscular dystrophy, diabetes, or other diseases. These sorts of discoveries begin to place true regenerative medicine within reasonable grasping distance.
Developmental biology also connects with understanding and controlling cancer. It was a genetic analysis in fruit flies that led eventually to the discovery of a lipid-decorated intercellular signaling protein called Hedgehog, which is also present in mammals, and the dissection of the signaling mechanism by which cells can detect and respond to it.
Hedgehog is multifunctional: it helps define the subtypes and spatial arrangement of neurons in many areas of the central nervous system, and the number and type of digits on the limbs. In some contexts, it controls the degree of cell proliferation, and we have found causative links between defective “always-on” signaling from the Hedgehog receptor and basal cell skin carcinoma.
Abnormal activation of other types of intercellular signals also has been found to be central to the development or progression of certain cancers. Among them: signals involving the “Wnt” family of related proteins that are, again, known to play similar roles in fruit flies and vertebrates.
There are many compelling examples to recite among the vast number of discoveries that are relevant to human disease and congenital problems. The articles in this issue cover several of them, hopefully conveying some of the sense of wonder and tremendous discoveries that have been and continue to be made.
I am reminded that it is a deep-seated scholarly drive that often provides a great stimulus toward determining, before anyone else, how a particular process works. Indisputably, some of the most telling discoveries relevant to human health were realized only after years of dedicated and tenacious scientific study. It is hard, however, to predict where the most translatable findings will come from next. In this field, perhaps more than in any other, we have learned over and over again that high quality basic research in esoteric or high-risk areas can often reap unexpected and large rewards.
Meanwhile, those of us in science hope to experience, more than once, that pure rush we feel when, looking down a microscope or learning of others’ findings in papers or at seminars, we suddenly see evidence of the totally unexpected way that nature runs things. In the end, the beauty and economy of biology always stand paramount.