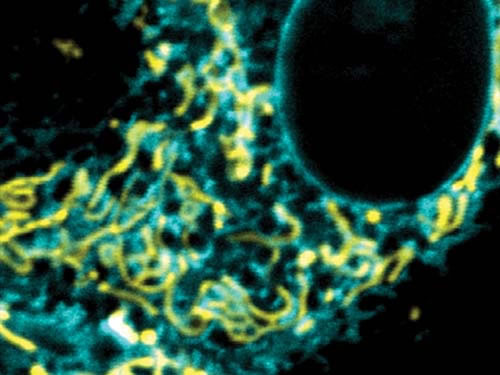
In 2002, the landmark Diabetes Prevention Program showed that moderate exercise and weight loss reduced by 58 percent the incidence of type 2 diabetes in people at high risk for developing the disease.
The problem is that few people have access to the intensive diabetes management provided to the study participants.
There also is growing evidence that some people are genetically susceptible to becoming obese and developing type 2 diabetes, and no matter how hard they try to lose weight, their bodies are working against them. “It’s extraordinarily difficult,” Rudolph Leibel, M.D., who co-discovered the fat hormone leptin.
A variety of medications, including those used to treat heart disease and lower cholesterol, can help prevent diabetes. But many of these drugs have side effects, and they don’t work in all patients.
Thanks in large part to the genetic and computer revolutions of the past 30 years, there has been a flood of new information about diabetes and obesity. Much of it at this point is incomplete. But progress is definitely being made.
First, a few definitions:
Glucose, a form of sugar primarily supplied by the diet, is a major fuel for the body and virtually the only source of energy for the brain. Because it’s so vital, extra glucose is stored in the liver and muscle in the form of glycogen. The liver also can make glucose from raw materials if an emergency supply is needed.
Insulin is secreted by “beta” cells, one of four types of cells that are clumped together in “islets” in the pancreas. One of its main jobs is to activate a pathway of biochemical signals that ultimately moves glucose from the bloodstream into muscle.
In the late 1970s, Daryl K. Granner, M.D., and his colleagues at the University of Iowa showed that insulin also turns down glucose production in the liver – another way of lowering blood glucose levels — by inhibiting the gene for an enzyme called PEPCK.
“This was the first example of insulin affecting a specific gene,” says Granner, the Joe C. Davis Professor of Biomedical Science at Vanderbilt University Medical Center and former director of the Vanderbilt Diabetes Center. “There are probably now 200 of those examples.” For instance, there is evidence that insulin acts on the brain to inhibit appetite and help regulate weight.
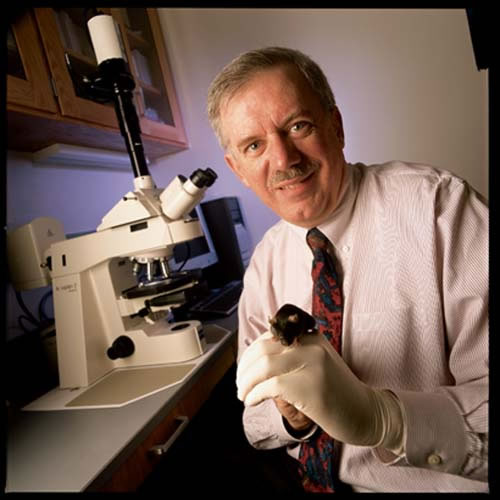
Photo by Dean Dixon
Other hormones are involved in the control of blood glucose. Glucagon, which is secreted by “alpha” cells in the islet, opposes the action of insulin and stimulates glucose production by the liver. So does cortisol, a stress hormone secreted by the adrenal glands on top of the kidneys.
This push-pull system is designed to keep blood glucose levels in balance, even after a feast, a fast or a flight from a predator.
Not enough blood glucose, a condition called hypoglycemia, can cause uncomfortable symptoms, including dizziness, confusion, heart palpitations and fatigue. It can lead to coma and death unless the glucose supply is replenished.
Too much blood glucose, the hallmark of diabetes, can damage the delicate inner lining of blood vessels, eventually leading to some of the major complications of diabetes – heart disease, kidney failure and blindness, caused by an overgrowth and leakage of tiny blood vessels in the retina of the eye.
“In type 2 diabetes there is an overproduction of glucose because these signals don’t work right,” says Alan D. Cherrington, Ph.D., holder of the Jacquelyn A. Turner and Dr. Dorothy J. Turner Chair in Diabetes Research at Vanderbilt who helped discover what glucagon does.
Constellation of symptoms
How do the beta cells know when to secrete insulin? Here’s part of the answer: They contain an enzyme called glucokinase, which puts a phosphate group on the glucose molecule. In the process, the enzyme stimulates the beta cell to secrete insulin.
Mark A. Magnuson, M.D., the Earl W. Sutherland Jr. Professor of Molecular Physiology and Biophysics who helped identify the role of glucokinase in insulin secretion, compares it to a “thermostat.” Depending upon whether it is blocked or activated, blood glucose rises or falls.
Maturity-onset Diabetes of the Young or MODY, a rare form of diabetes, can result from mutations in any one of at least six different genes that play a role in the ability of the beta cell to sense the presence of high levels of glucose, including the gene for glucokinase.
“Type 2 diabetes … may actually be a disease of a disordered thermostat,” says Magnuson, who chairs the steering committee of the Beta Cell Biology Consortium, an effort supported by the National Institute of Diabetes and Digestive and Kidney Diseases to improve understanding of the beta cell.
The challenge is to develop drugs or other treatments that can adjust the thermostat without causing serious side effects.
“That’s why (diabetes), despite all the investment of money, intellect and resources, is still getting worse,” says Cherrington, former chairman of the Department of Molecular Physiology and Biophysics at Vanderbilt and past president of the American Diabetes Association. “As yet we don’t understand the complexity of the disease.”
As an example of that complexity, an estimated 17 million Americans have diabetes, but nearly four times that number may have metabolic syndrome, also called insulin resistance, a constellation of symptoms that places them at high risk for developing type 2 diabetes and heart disease.
Symptoms include abdominal obesity, high blood pressure, high blood glucose, high levels of trigylcerides and low levels of high-density lipoproteins (HDL). Trigylcerides are a form of fat that contribute to atherosclerosis, the build-up of fatty deposits in blood vessels, whereas HDL is a type of cholesterol that seems to protect against it.
Fat cells, also called adipocytes, also can build up in the liver and pancreas, affecting their ability to function properly. In addition, recently discovered hormones released by adipose tissue, including leptin, resistin and adiponectin, can influence glucose metabolism in one way or another, some by opposing or complementing the effects of insulin, others by signaling the brain to suppress appetite. So can hormones released the digestive tract, such as ghrelin, PYY and GLP-1.
“You cannot study the beta cell in isolation,” explains Christopher B. Newgard, Ph.D., director of the Sarah W. Stedman Nutrition and Metabolism Center at Duke University. “There is a remarkable interplay and dovetailing of these regulatory circuits.”
How are scientists able to tease out the elements of this complex network of interactions? Here are some of the ways:
Gene microarrays
The recent sequencing of the human genome (and those of other animals) is just the latest in a series of critical advances during the past quarter century that have enabled scientists to identify specific genes, map their location and determine what they do.
In just the past three or four years, researchers have figured out how to monitor the expression of thousands of genes simultaneously by spreading complementary bits of genetic material across a glass slide, called a microarray. Active genes will bind to the array in a way that can be can be detected by fluorescence and other tagging techniques.
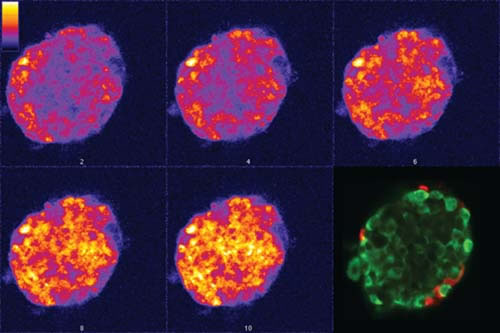
“We’re not after single genes anymore,” explains Leibel, head of the Division of Molecular Genetics and co-director of the Naomi Berrie Diabetes Center at Columbia University. “We’re after the interaction of multiple genes … We’re trying to study the whole biology.”
Thanks to a recent initiative supported by the National Institute of Diabetes and Digestive and Kidney Diseases, researchers at Vanderbilt and elsewhere are creating pancreas-specific microarrays – chips the size of a saltine cracker that contain all of the genes expressed over the life span of the beta cell in mice and humans. This method allows scientists to determine which genes are turned “on” or “off” in pancreatic cells, and it has aided the discovery of previously unidentified genes that play a role in the development of pancreatic islets, Magnuson says.
In vivo imaging
The secret life of the beta cell is being revealed. Thanks to recent advances in laser microscopy, scientists are able to study the “behavior” of beta cells in pancreatic islets grown in the laboratory, and even in animals.
At Vanderbilt, for example, David W. Piston, Ph.D., has helped pioneer the use of fluorescence imaging methods to trace the sequence of events, beginning with the entry of glucose into beta cells, that ultimately leads to insulin secretion.
A goal is to figure out why, in type 2 diabetes, beta cells are unable to respond normally to blood glucose, and what might be done to “fix” that problem. “It’s hard to learn these things without looking in the intact animal,” says Piston, professor of Molecular Physiology & Biophysics and Physics.
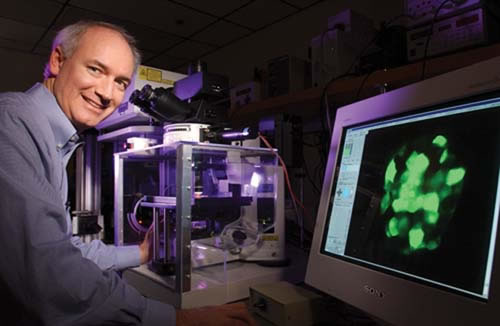
The techniques, which include two-photon excitation microscopy, also enable Piston to watch mouse islets grow a new blood supply when they are transplanted into another part of the body. This information could lead to improvements in islet transplantation as a treatment for type 1 diabetes.
“Islets are really a cellular transplant as opposed to an organ transplant. They have to develop new blood vessels,” explains Alvin C. Powers, M.D., director of the Vanderbilt Diabetes Center, who is working with Piston on the project. “How do cells that are transplanted survive, and how can you improve that process?”
Whole animal studies
Many studies of how glucose is produced or used have been conducted in dogs because their blood vessels are large enough to allow detailed studies of glucose, insulin and all of the other factors that play a role in the disease.
At Vanderbilt, Masakazu Shiota, Ph.D., assistant professor of Molecular Physiology & Biophysics, has miniaturized a method for measuring insulin sensitivity in muscle so it can be applied to rats and mice, which are easier to handle. The technique enables researchers to measure how much radiolabeled glucose is absorbed by muscle in response to a continuous infusion of insulin.
These studies are helping researchers determine how glucose gets into the muscle cell, and what may be going on when tissues become resistant to insulin, says David H. Wasserman, Ph.D., director the federally funded Mouse Metabolic Phenotyping Center at Vanderbilt. The center, one of four in the country, helps develop new mouse models of diabetes to further research in the disease.
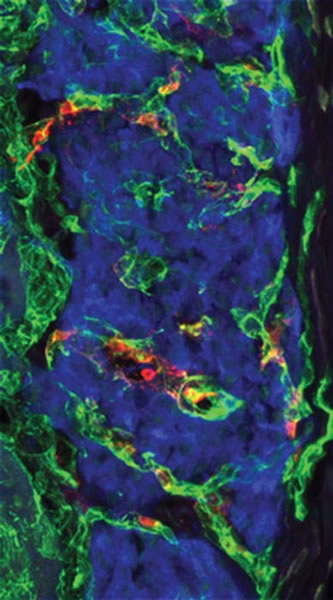
Animal studies also are shedding light on the complications of the metabolic syndrome. Two years ago, for example, Drs. MacRae Linton and Sergio Fazio at Vanderbilt and their colleague, Gokan Hotamisligil at Harvard found that they could protect mice from atherosclerosis, the build up of fatty deposits in blood vessels, by eliminating a protein linked to insulin resistance.
The adipocyte fatty-acid-binding protein (aP2) is produced by adipocytes (fat cells), which contribute to insulin resistance, and also by macrophages, inflammatory cells that play a crucial role in atherosclerosis.
The researchers studied apoE-deficient mice, a widely used mouse model of high cholesterol and atherosclerosis. Mice that lacked aP2 were dramatically protected from atherosclerosis, even though they remained insulin resistant, suggesting that the expression of aP2 by macrophages promotes atherosclerosis.
This finding was confirmed in the following way: apoE-deficient mice lacking expression of aP2 only in macrophages were similarly protected from developing atherosclerosis. Earlier studies found that obese mice lacking aP2 expression in adipose tissue showed increased insulin sensitivity. Thus, aP2, which links two major features of the metabolic syndrome, is a potential drug target for preventing both atherosclerosis and insulin resistance.
Viral vectors as Trojan horses
One of the new tools in the researchers’ armamentarium is the recombinant adenovirus, a common respiratory virus that has been genetically engineered so that it is relatively harmless.
In 2003, researchers at Baylor College of Medicine in Houston and Shiga University in Otsu, Japan, reported they had used this tool to completely reverse diabetes in mice that had high blood glucose because of non-functioning beta cells.
The researchers inserted genes for beta cell growth and transcription factors into the genetic material of the adenovirus. After injection into the bloodstream, the viral “vector” traveled to the liver. There, the imported genes, also called “transgenes,” expressed factors that seemed to stimulate formation of new, insulin-secreting beta cells and reversed the hyperglycemia.
While more study of these “transgenic” mice is needed before the technique can be attempted in humans, this finding, reported in the journal Nature Medicine, raises hopes for a gene therapy that could reverse type 1 diabetes in humans.
Adenovirus vectors also have proven useful in exploring the relationship between obesity and insulin resistance.
For example, Newgard and his colleagues recently tested an adenovirus vector that contained the gene for malonyl-CoA decarboxylase, an enzyme that essentially can “melt fat” out of tissue. The vector was used to carry extra copies of the gene into the livers of rats that had become insulin resistant after being fed a high-fat diet.
The researchers found that when the enzyme was over-expressed and specifically melted fat out of the liver, insulin sensitivity in the muscles improved.
“Somehow melting the fat out of the liver is a signal to restore insulin action in the muscle,” Newgard says. “So there’s tissue networking going on (messages sent between organs and tissues) that we are only at the threshold of understanding.”
Knock-out mice
Another important research tool pioneered by Magnuson and his colleagues at Vanderbilt involves the use of “tissue-specific knock-out” mice – animals in which a gene has been inactivated in a specific tissue like the liver or pancreas by an enzyme called Cre recombinase.
In 1999, for example, Magnuson, C. Ronald Kahn, M.D., former president of the Joslin Diabetes Center in Boston, and their colleagues discovered that beta cells have their own functional insulin receptors. When these receptors are “knocked out,” the beta cells don’t secrete insulin normally in response to a rise in blood glucose.
This finding suggests that insulin can regulate its own secretion and that impaired regulation may contribute to inadequate insulin secretion that is a hallmark of type 2 diabetes.
“There is nothing that we’ve found that is so precise, so accurate and so useful as that (Cre) enzyme,” says Magnuson, who also directs the Vanderbilt Center for Stem Cell Biology. “It is like a cruise missile for manipulating the mouse genome.”
“We are in a truly golden era of biomedical research,” adds Kahn. “The technologies and the insights we have into basic biology and genetics and biochemistry are so great and so powerful that we can do almost any experiment that we think about.
“What is limiting in many cases are both the monetary and the human resources to do that … The number of people training in pediatric diabetes and endocrinology is very small considering the number of young people now who are developing not only type 1 diabetes but also type 2 diabetes and obesity.”
Another pressing need is public education and other measures to stem the rising tide of obesity and diabetes.
“The Human Genome Project will not solve the obesity and diabetes epidemic,” asserts Frank Vinicor, M.D., MPH, director of diabetes translation for the U.S. Centers for Disease Control and Prevention. “We’re not going to find a magic pill … We have to start addressing primary prevention or we’re going to be overwhelmed.”
“Ultimately to prevent obesity, diabetes and high blood pressure, we’re going to have to get to the children,” adds David G. Schlundt, Ph.D., associate professor of Psychology at Vanderbilt who is involved in local diabetes prevention efforts. “Once you’re 45 years old and weigh 300 pounds and have high blood pressure and diabetes, there’s only so much that can be done.”