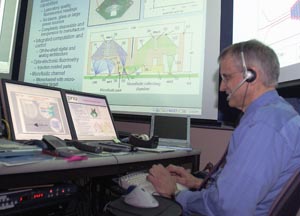
John Wikswo, Ph.D., at work in the vibro-acoustics lab at Vanderbilt University.
photo by Daniel Dubois
$5.5M grant to boost bio-weapons detection
Developing a device that will help security forces respond to possible chemical or biological terrorist attacks is the object of a new $5.5 million, five-year collaboration between scientists at Vanderbilt University and the U.S. Army Edgewood Chemical Biological Center at the Aberdeen Proving Ground, Md.
The Centers for Disease Control and Prevention maintain a “select list” of more than 70 biological agents. These are deadly toxins, viruses and bacteria that have been identified as those which terrorists are most likely to use in their attacks. The government has mounted a major research effort to develop detectors that can identify these agents and to come up with antidotes and treatments for them.
There are several scenarios, however, that could render much of this effort irrelevant. For example, terrorists could:
• Develop and use unknown toxins or biological agents.
• Use genetic engineering to turn harmless agents into deadly toxins.
• Use genetic engineering to alter known biological agents so they can't be identified.
• Hide toxins in complex chemical cocktails that confound and mask their detection and treatment.
The new device being developed is called a “NanoPhysiometer” and it takes the old “canary in the coal mine” trick and gives it a new, high-tech twist.
The basic idea is to create nanoscale cages small enough to hold a single cell up to a few thousand cells, add an array of biosensors that can monitor the cells' health and then expose them to minute quantities of suspected biological agents.
According to theory, the cells should respond within seconds to anything harmful, and the way in which they react should provide clues to the nature of the unknown agent that can help identify effective treatments and forms of prophylaxis.
“Cells are the ultimate canary,” says Roy Thompson, research biologist at the Edgewood Chemical Biological Center and a key participant. “They respond to a wide range of chemicals and biologicals and signal from one cell to another about what's going on in their environment — that's their role. Using whole cells allows you to detect cellular signatures for a broad range of chemical and biological agents.”
According to principal investigator John P. Wikswo, Ph.D., director of the Vanderbilt Institute for Integrative Biosystems Research and Education (VIIBRE), the approach “is extraordinarily versatile” because it measures the biological impact of the toxins, not just their presence.
However, developing such a device demands a truly interdisciplinary research effort that involves physicists, engineers, chemists, biologists, mathematicians, physiologists and toxicologists.
Thompson is the project's connection to the U.S. Department of Defense's effort to develop better sensors and other effective countermeasures against chemical and biological warfare agents.
“The requirements for environmental monitoring for the health of the soldier and the health of the civilian population have expanded tremendously, given 9/11 and the fears of chemical and biological terrorism,” he says.
Assistant Professor of Chemistry David Cliffel, Ph.D., has been working with Thompson for several years to develop the big brother and predecessor of the NanoPhysiometer.
Cliffel's research group created the bulky laboratory instrument, called a “multianalyte microphysiometer,” by modifying a commercial device called a Cytosensor that was designed to measure changes in acidity (pH) in small chambers that hold between 100,000 to 1 million cells.
Cliffel's group added three additional sensors, allowing the instrument to simultaneously measure the concentrations of the key compounds that cells consume and excrete — oxygen, glucose and lactic acid — in addition to pH. It takes these measurements every few minutes, which allows the researchers to monitor the health of the cells in near real time.
Two years ago, Cliffel provided Thompson with a multianalyte microphysiometer for use in the Edgewood Laboratory, which is designed specifically to handle deadly toxins. This has allowed them to study how small populations of cells react to the presence of agents like anthrax, ricin and botulinum toxins. One of their goals has been to identify changes in cell metabolism that can quickly identify each type of toxin.
At the same time, Franz Baudenbacher, Ph.D., assistant professor of Biomedical Engineering and Physics, has been pursuing the challenging task of shrinking the multianalyte microphysiometer down to the size of a computer chip. Baudenbacher's group has developed the capability to capture, manipulate, grow and study single living cells in extraordinarily small containers with volumes barely larger than the cells themselves. Liquid flowing through tiny channels carries individual cells from place to place, provides the cells with nutrients and carries away their wastes. The researchers are also miniaturizing the biosensors that detect the cells' activity to the point where they are less than one-fifth the width of a human hair.
Baudenbacher's team has developed a method for making such devices out of a silicone polymer similar to that used in soft contact lenses and they have developed a pH biosensor that is small enough for the application.
In a recently published study they reported that they were able to keep single mouse heart cells alive for several hours in such a device, that they could deliver a drug to a portion of the cell and that they could produce an “electrocardiogram” for a single cell. This signal plays a particularly important role in the functioning of heart and nerve cells.
Jacek Hawiger, M.D., Ph.D., Oswald T. Avery Distinguished Chair and Professor of Microbiology and Immunology, and Owen McGuinness, Ph.D., associate professor of Molecular Physiology and Biophysics, bring a wealth of biological expertise to the project.
Hawiger and his colleagues in the department of Microbiology and Immunology study the cellular and immunological responses to a variety of biological toxins, including staphylococcal enterotoxin B, anthrax toxins and botulinum neurotoxins.
McGuinness is an expert in cellular metabolism with a long-standing interest in how biotoxins affect metabolic processes.
He describes the output of the new sensors as “metabolic fingerprints,” which the team hopes will be unique for different toxins and provide the key for their rapid discrimination and identification.
McGuinness and Hawiger will participate in understanding and deciphering what the metabolic fingerprints really mean and in determining what additional pieces of information will make them more meaningful.
Since 2001, the VIIBRE group has been pursuing such foundational research with support from a $1.9 million grant from the Defense Advanced Research Project Agency.
The goal of the project, which has been met, was to demonstrate that detection systems based on sensing the response of whole cells can provide rapid detection of toxins and could be useful in drug screening efforts.
The ultimate goal of the collaborative program is to produce a compact device that soldiers can carry into the battlefield and first responders can carry to the site of a terrorist attack that can monitor the environment for unknown biological or chemical agents.
In addition, when the presence of a bioagent is detected, the portable device can provide information about a toxin, even before it has been identified, that can help identify the drugs or antidotes that are best suited for treating those who have been exposed.
Other key participants in the current project are Assistant Professor of Mechanical Engineering Mark Stremler, Ph.D., and Research Assistant Professor of Physics Momchil Velkovsky, Ph.D.