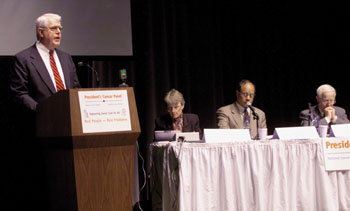
Dr. Clifton Meador, executive director of the Meharry-Vanderbilt Alliance, described the innovative initiative to the President's Cancer Panel. The panel included, from left, Maureen Wilson, Ph.D., Dr. Harold P. Freeman, and Dr. Paul Calabresi. (photo by Dana Johnson)
DNA repair proteins examined
To repair damaged DNA, cells employ entire teams of protein mechanics–alternating team members to handle different kinds of repair problems. Center for Structural Biology researchers studying DNA repair reported in a recent issue of Cell that a protein called “replication protein A” could act as a coach, assembling team members and coordinating plays.
“Our DNA is constantly being challenged and damaged by things that are naturally and unnaturally in the environment,” said Walter J. Chazin, Ph.D., Chancellor’s Professor of Biochemistry and director of the Center for Structural Biology. “It is the critical job of DNA repair machines to correct any mistakes and prevent cancer-causing mutations.”
Chazin’s group focused on replication protein A because it is abundant in the cell, especially in the nucleus, and it is part of the machinery for several different DNA handling processes.
“Replication protein A is a very rich protein to study, because it represents an intersection point. It is a molecule that allows us to look at the interactions and parallel universes of DNA replication, recombination, and repair,” Chazin said.
Replication protein A is suited to a role in these processes because it can interact simultaneously with DNA and various proteins. Previous work had demonstrated that a DNA repair protein called XPA binds to replication protein A.
In the current work, Chazin and colleagues carefully determined the exact interacting region of XPA and then looked for a similar region in proteins responsible for other kinds of DNA repair. Using a structural biology tool called NMR (nuclear magnetic resonance) spectroscopy, they showed that XPA and two other DNA repair proteins bind to replication protein A in exactly the same way.
The findings suggest that replication protein A is more generally involved in multiple different types of DNA repair, Chazin said.
The structure of the binding area turned out to be a flat sticky surface with complementary positive and negative charges. These features determine the recognition between the two proteins. A flat binding surface differs from the tight protein interactions referred to as “lock and key” or “ball and joint” interactions, Chazin said.
“What’s beautiful about this finding is that a flat surface fits very well with having a lower affinity interaction, and that’s exactly what you need in these multi-step processes,” he said.
Because DNA repair involves up to 12 different proteins acting on the site of damage, it is important that the order of their activity be precisely coordinated. Replication protein A may function as a coordinator of a “hand-off” process in which repair proteins bind, do their jobs, and get handed off so the next proteins can come in to do their jobs, Chazin said.
“This is a really nice example where a ‘hand-off’ mechanism makes perfect sense,” he said. “It’s important to be able to understand how the ‘hand-off’ works at the atomic level because we will be seeing this again and again in other multi-protein machines.”
Chazin and colleagues will continue to explore how replication protein A and the other protein parts of the DNA repair machine work to accomplish their complex tasks. They will take advantage of the powerful capabilities of the new Vanderbilt Biomolecular NMR Center, which Chazin helped to design and now directs.
Investigators participating in the research published in Cell were Georges Mer, Alexey Bochkarev, Rajesh Gupta, Elena Bochkareva, Lori Frappier, C. James Ingles, and Aled M. Edwards. The research was supported by the National Cancer Institute of Canada, the National Institutes of Health, and the National Science Foundation.