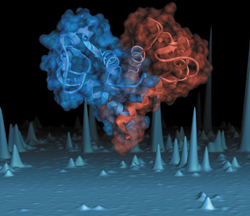
This image, which appeared on the cover of Nature Structural Biology, shows a combined ribbon diagram and molecular surface representation of the atomic resolution structure of calcyclin, a protein that is found in very high abundance in patients with acute myeloid leukemia.
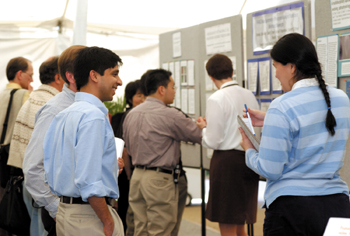
Maria Person, Ph.D., from the University of Texas at Austin, talks with Ashutosh Mishra of St. Jude Children's Hospital during a poster session at the Proteomics Conference last week. (photo by Dana Johnson)
by Bill Snyder, Mary Beth Gardiner and Leigh MacMillan
After sequencing the human genome, the next “grand” biological challenge is determining the function of proteins and the complex networks they form to carry out nearly all the tasks of life, from building bone and muscle to carrying signals through the brain.
This field of scientific inquiry, called “proteomics,” is critical to understanding both health and disease, and it was the subject of a national conference May 19-22 at Vanderbilt University School of Medicine. More than 200 scientists from throughout the world attended.
The keynote speaker was Dr. Leroy Hood, who is nationally known for his scientific innovation and entrepreneurial spirit. Hood played a major role in developing the technology for sequencing the human genome, and he has helped create a number of biotech companies. He recently founded the Institute for Systems Biology in Seattle, Wash., which aims to understand how genes, proteins and other factors work together in complex biological systems.
Scientists hope this information will lead to the development of more effective drugs with fewer side effects, and to the ability to diagnose and prevent disease before it starts. “We’re on the threshold of the journey to cure disease,” said Richard Caprioli, Ph.D., Stanley Cohen Professor of Biochemistry, who co-directed the conference with Walter J. Chazin, Ph.D., Chancellor’s Professor of Biochemistry and Physics.
It won’t be an easy journey, Caprioli added. That’s because there are 500,000 or more proteins in the human, compared to an estimated 35,000 genes. And whereas genes are relatively constant, proteins are “molecular machines in motion,” often traveling within and between cells, changing their function and interacting with other proteins in complex ways.
Caprioli, who directs the Mass Spectrometry Research Center at Vanderbilt, chaired the first session of the conference, which focused on the technologies that are evolving to facilitate the study of proteins. A pair of presentations on mass spectrometry provided a glimpse of how this particular tool, which analyzes proteins based on properties related to molecular weight, has been modified to better identify the proteins within a cell or, in conjunction with imaging technology, to analyze protein distribution in tissue.
Donald F. Hunt, Ph.D., University Professor of Chemistry and Pathology at the University of Virginia and a longtime innovator in the field, described how tandem mass spectrometry can be used to discriminate single proteins from a pool of thousands at levels of extreme sensitivity.
Modifications of the basic technology, such as ion-trap mass spectrometry and the use of Affymetrix DNA chips as an adjunct, increase sensitivity further, allowing detection of minute amounts — from five attomoles to 100 femtomoles — of protein. In one project comparing surface proteins in cultured breast cancer cells versus normal cells, the technology successfully identified five proteins that were unique to cancer cells, from a pool of 10,000.
Imaging mass spectrometry is a modification developed by Caprioli and his colleagues, Markus Stoeckli, Ph.D., and Pierre Chauraud, Ph.D., which provides a “molecular photograph” of a tissue. Caprioli explained how the MALDI-TOF (Matrix-Assisted Laser Desorption Ionization – Time of Flight) spectrometer can be used to directly evaluate discrete areas of a tissue section, or for more global analysis of a tissue specimen. The technology also is being tested as a way to predict the progression of disease and monitor drug efficacy.
Other methods are being developed to study how proteins interact with each other. Roger Tsien, Ph.D., professor of Pharmacology, Chemistry and Biochemistry at the University of California, San Diego, reported how changes in the color of fluorescent labels can be used to study protein interactions inside a living cell.
Understanding how proteins interact is key to the development of new drugs, said Chazin, director of the Center for Structural Biology. An example is the development of drugs that block an enzyme essential for the production of the human immunodeficiency virus, which causes AIDS. “Those were based on the fact that we were able to determine three-dimensional structures of the (enzyme), and from that we went to molecular modeling and designed small molecules. They proved to inhibit the enzyme very effectively… and now are in use in the clinic,” he said.
Computer-based molecular modeling techniques can predict the structure and function of proteins based on the sequence of genes that encode them. They also may allow scientists to change the structure in order to “improve” the function of the protein. “For example, you might imagine making a variant of insulin which is more active,” said Steve Mayo, Ph.D., associate professor of Biology and Chemistry at the California Institute of Technology. “You might then have to take it less frequently.”
One of the promising new proteomics technologies is protein chips-postage stamp-sized grids of tiny dots, each dot a different protein. The thousands of proteins dotting the surface of a glass slide, a single protein chip, can be probed to ask questions about protein-protein and protein-drug interactions, said two pioneers of the technology, Gavin MacBeath, Ph.D., assistant professor of Chemistry and research fellow at Harvard University’s Bauer Center for Genomics Research, and Michael Snyder, Ph.D., chairman of Molecular, Cellular and Developmental Biology at Yale University.
By looking for protein-protein interactions using protein chips, investigators can begin to understand the networks of interactions that regulate cell function. Protein chips, or microarrays, follow on the heels of DNA microarrays, which in the last several years have become a powerful tool to study gene expression. If the technology of DNA microarrays is “awesome,” Snyder said, “we think protein microarrays should be really awesome.”
Snyder’s group has created protein chips that include nearly all of the more than 6,000 yeast proteins. To illustrate the usefulness of these “yeast proteome chips,” Snyder described an experiment to detect proteins that interact with the protein calmodulin. Calmodulin interacted with 45 proteins on the chip-12 proteins that were already known or suspected to bind it, and 33 new targets. “Every time you do these experiments, you find very interesting results that you did not expect,” he said.
Finding unexpected members of protein complexes — made possible by high throughput proteomics approaches — was the theme running through the conference’s final scientific session.
Andrew Link, Ph.D., assistant professor of Microbiology and Immunology at Vanderbilt, described a novel method for directly analyzing the proteins within a large complex. He and collaborators have used the technique to study complexes of proteins that copy the DNA message into RNA and those that manufacture proteins. Even in these well-studied complexes, Link said, “we still found new proteins.” The challenge, he said, is what to do with the lists of proteins and reams of data that are generated.