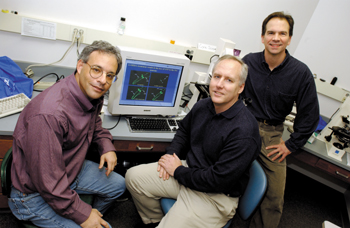
Leading the Vanderbilt University Medical Center research is, from left, Richard Nass, Ph.D., Randy Blakely, Ph.D., and David Miller III, Ph.D. (photo by Dana Johnson)
Worm research yields powerful model for Parkinson’s disease
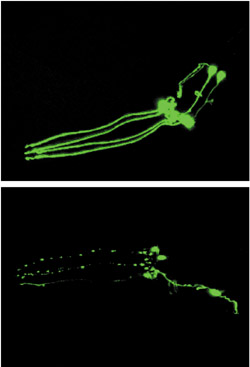
Images showing impact of 6-OHDA on dopamine neurons in C. elegans. The top frame shows intact green-fluorescing dopamine neurons in an untreated C. elegans worm. The bottom frame shows a similar worm three days after exposure to 6-OHDA. The partial loss of green fluorescence is indicative of progressive death of the dopamine neurons.
It was a typical Tuesday night at the lab when Richard Nass, Ph.D., walked down the tiled hallway and entered a small, darkened room. Settling himself onto a stool, he placed a shallow dish on the stage of the microscope before him and peered into the eyepieces. What he saw—or, rather, didn’t see—took him aback.
Nass saw the host of wriggling, transparent worms that he expected, but missing was the distinctive green glow that should have lit up the bodies of the worms like neon.
“I almost couldn’t believe it,” he said, shaking his head.
The loss of green fluorescence that Nass observed in his worms told him that their dopamine neurons, which had been genetically altered to fluoresce green, had been destroyed by exposure to a chemical, 6-hydroxydopamine (6-OHDA). The results of the study, published this week in the journal Proceedings of the National Academy of Sciences, suggest that this tiny roundworm, named C.elegans, can serve as a powerful model for studying the molecular mechanisms underlying degeneration of dopamine neurons in the brains of patients with Parkinson’s disease (PD).
The paper, co-authored by Nass, a post-doctoral research fellow in Pharmacology; Randy D. Blakely, Ph.D., Alan D. Bass Professor of Pharmacology; and David M. Miller, III, Ph.D., associate professor of Cell Biology, presents evidence for why and how the C. elegans model can be used to visually screen for genetic and environmental events that influence dopamine transporter expression and trigger dopamine neuron cell death.
Although rare genetic forms of PD have been identified, the molecular basis of dopamine neuron vulnerability and cell death in the majority of PD cases is poorly understood. Some theories on the cause involve exposure to environmental toxins, the generation of reactive oxygen species in brain cells, and inhibition of mitochondria, the subcellular structures responsible for energy production in the cell. How these events might selectively target dopamine neurons is unresolved.
The chemical Nass used in his experiment, 6-OHDA, is only slightly different from dopamine, the chemical normally accumulated by the dopamine neuron, but when 6-OHDA is transported into dopamine neurons, it can produce destructive reactive oxygen species.
“Just one small addition of an oxygen and a hydrogen in just the right place in that molecule renders it very, very reactive in causing oxidative damage to the cell,” Blakely said.
Even more intriguing, 6-OHDA has been found in brain and urine samples of PD patients, suggesting it might somehow participate in the development of PD.
The investigators were led to the use of C. elegans as a model for PD by an indirect route. The Blakely lab had for some time been studying the dopamine transporter, intrigued by how certain drugs, such as cocaine and Ritalin, block absorption and transport of dopamine in mammals. A few years back, Blakely joined forces with Miller, an established C. elegans neurobiologist, to clone the worm’s dopamine transporter gene. They found that the dopamine transporter in C. elegans is essentially the same, structurally and genetically, as the one found in mammals, but much easier to study because of the nature of the organism.
C. elegans is a small nematode, only one millimeter in length, that is popularly used in neurological research because of its short lifespan and its transparent body, which allows for direct visual assessment of neuronal structures. The worm has about 300 neurons in its nerve system, eight of which specialize as dopamine neurons. Nass devised a way to make these eight cells glow green when viewed through a special microscope, and the three dimensional effect is striking. As the worm rolls and twists, the luminous network of threadlike green neurons running through the head and trunk of the worm is easy to discern.
“We thought initially that we could develop a behavioral test—that we might manipulate these dopamine neurons and the worms would behave a certain way,” Blakely said. “But David, Richard, and I were worried because these neurons in worms contribute to some fairly subtle behaviors. After Richard made this line of worms having the glowing green neurons, we realized the thing we ought to do is to take advantage of this highly visible marker.”
Nass found that exposing his worms to 6-OHDA caused the dopamine neurons to die—and the green lights to go out. Blocking dopamine transporter function through drugs or by genetic disruption eliminated 6-OHDA-sensitivity in the worms—in this case, the green lights were not extinguished. These results suggest that the toxic effect of 6-OHDA occurs after it enters the dopamine neuron.
The effect of the neurotoxin is rapid. The dopamine transporter sucks up the 6-OHDA like a micro-vacuum cleaner, and within an hour the green lights begin to dim. After 24 to 48 hours, death of the dopamine neurons is complete and the green glow has disappeared entirely.
To confirm that the loss of fluorescence was really due to death of the neurons and not a quenching or bleaching effect, Nass knew he needed to take a closer look at the worm using electron microscopy techniques. Miller referred Blakely and Nass to his collaborator, David H. Hall, Ph.D., a faculty member at the Albert Einstein College of Medicine and expert in C. elegans anatomy. Nass traveled to New York and, for nearly a month, helped prepare hundreds of ultrathin sections of his 6-OHDA-treated worms for electron microscopy. He photographed and evaluated the electron micrograph image of each section, and found that, indeed, the dopamine neurons were damaged.
“That was a great experience,” Nass said. “Each week, I arrived at the lab on Monday and left on Thursday, working almost non-stop, even sleeping in the lab to avoid time lost to commuting. David Hall said I did a year’s worth of work in the time I was there.”
Nass and Blakely believe that the development of this model will expedite research into the cause of PD. According to Nass, the power of the model system is that no assumptions as to specific genes or pathways need to be made to identify candidate genes involved with degeneration, and that the same system used to understand the mechanism of toxicity can support a screen for protective agents.
“Unlike in much of science where you have something in mind in advance, such as how a specific protein is involved in regulation,” he said, “here we can randomly mutate and see right away which animals are completely resistant to the toxin. From there, we can quickly identify the gene responsible for that protection.”
Blakely envisions the model as a way to screen chemical libraries for small molecules to identify candidates for drug development.
“This is being done in other organisms—in zebrafish and even in mice— but it’s a fairly difficult, slow, and costly process,” he said. “We think we can do that in the worm more efficiently, and that’s one of our future goals.”
To expand their ability to visualize the effects of chemical exposure in their model, the team also foresees introducing a second color into their transgenic worms.
“We are developing the use of different colors of reporter proteins simultaneously, so we can look at more than one structure at a time, such as a second type of neuron,” Miller said. “And since we don’t know what subcellular structures are targeted initially by the toxin, we could mark specific ones—such as mitochondria—and observe the effect.”
Nass’s project, which was funded largely by the National Institute for Diabetes & Digestive & Kidney Diseases, was part of a larger research effort between four labs at VUMC that became a program project grant, which is headed by Kevin Strange, Ph.D., professor of Anesthesiology and Pharmacology, and includes Blakely, Miller, and Dr. Al George, professor of Pharmacology and Medicine and director of the Division of Genetic Medicine.
Nass received independent support from a Neuroscience Training Grant and an award from the Pharmaceutical Research and Manufacturing Association (PHARMA). The current project is also supported in part by funds from the National Parkinson Foundation.
The surprised delight Nass felt looking into the microscope that summer night was out of recognition that, though millions of years of evolution separate this simple worm from man, the mechanism behind 6-OHDA toxicity in dopamine neurons might very well be the same. It was, he said, the kind of moment that “drives you to do science.”
“It was a real thrill for me,” he said. “Knowing the types of studies done in mammalian systems—in monkeys and others—and, in that instant, seeing a similarity between this small guy you can barely see with the naked eye and those higher animals, well, it was just really remarkable.”