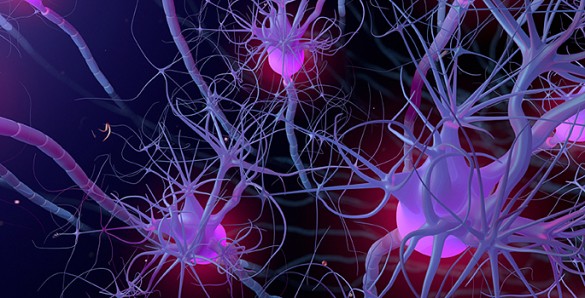
by Emily Poulin
A new light-based technology is allowing scientists to peer deep into the brain and alter animal behavior with the “flip of a switch.”
Called optogenetics, for optics and genetics, the technology harnesses the power of light-activated ion channels from algae and combines it with genetic techniques to study isolated neuron populations.
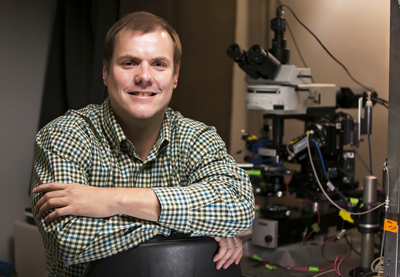
“It’s an incredibly powerful tool…probably the No. 1 technique in the past 10 years,” said Brad Grueter, Ph.D., assistant professor of Anesthesiology at Vanderbilt. His lab is using optogenetics to study the nucleus accumbens, a brain region involved in addiction-related behavior.
A momentous challenge facing neuroscientists is isolating the particular neuron population that mediates a behavior from the dense neuronal network that makes up the brain, a challenge that can now be surmounted using optogenetics.
Traditional means of determining whether a particular brain region was associated with a behavior involved electrically stimulating the region and evaluating whether that behavior was altered.
“Before, you could say this region was involved in this, and that region was involved in that, but all these are heterogeneous regions with lots of cell types. Optogenetics allows you to go in and ask about a specific type of cell,” said Danny Winder, Ph.D.
Winder, professor of Molecular Physiology and Biophysics, and his Vanderbilt colleagues are using optogenetics to study which neurons control stress-induced relapse in addiction.
For the first time, using optogenetics, neuroscientists have fine-tuned spatial and temporal control over specific neuron populations. The key? Employing a class of light-activated ion channels from algae, called channelrhodopsins.
Using genetic techniques, scientists are able to insert channelrhodopsins into the exact neurons they are interested in. Upon exposure to specific wavelengths of light, the channelrhodopsin opens and the neuron is activated.
In practice, optogenetics allows control over neurons by simply turning a light on and off, allowing researchers to test the effects of activating specific neurons in a direct way.
“With electrical activity, you don’t know for sure that the neuron is firing because you’re not necessarily driving it,” said Jeff Jones, a graduate student in the lab of Douglas McMahon, Ph.D., Stevenson Professor and chair of the Department of Biological Sciences.
“With optogenetics, you’re directly activating a neuron through the ions flowing into the cell itself,” Jones said.
With optogenetics, the precise identification of a neuron population responsible for an output can be identified, a layer of specificity that has not existed until now. “Before, it was all correlative,” Grueter said. “We can now test causality.”
When applied at a whole animal level, a mouse’s behavior can be altered with a simple light switch, almost like having “remote-controlled mice,” he said.
Neurons are connected to each other like an electrical circuit that relays information all over the brain. Understanding how the circuitry is wired may open a window into the origin of different behaviors.
Sachin Patel, M.D., Ph.D., associate professor of Psychiatry, is using optogenetics to map the circuitry of the “fight or flight” response, generated in certain neurons of the amygdala.
“Using this technique we’re able to ask: what are the types of drivers that elicit activity in these neurons and then, what are the downstream targets of these neurons?” Patel said.
Optogenetics allows this wiring schematic to be examined at multiple levels. Several channelrhodopsins have been commandeered from algae, some which are activated by different wavelengths of lights. With this strategy, one neuron can be engineered to express a blue light-activated channelrhodopsin, and another neuron a green light-activated channelrhodopsin.
Using multiple light sources, researchers can now observe interactions between different neuron populations.
Graduate student Michael Tackenberg is working with Jones in McMahon’s lab to study the effects of manipulating circadian rhythms, the biological clock that keeps us running on a 24-hour cycle. Their research capitalizes on the temporal control that optogenetics offers.
“We’re talking about very quick changes that happen and changes that happen repeatedly,” Tackenberg said. “Using optogenetics we’re able to replicate physiological events better than we could with, say pharmacological techniques.”
With traditional means, “the transitions from on to off would be very slow and fairly unpredictable,” he continued. “With this, it’s as fast as the light turns on.”
The implications for human health can be extrapolated, but for now are limited by the ability to get channelrhodopsins into a human patient.
The first future application that comes to mind is as a replacement for deep brain stimulation, which is used to treat multiple disorders, including Parkinson’s disease.
Deep brain stimulation involves electrical stimulation of the brain to relieve the symptoms of Parkinson’s, “but there’s a lot of gray area about what exactly is being stimulated and what else is being stimulated that’s producing unwanted side effects,” Winder said.
“If you had a way of isolating the pathway that really needs to be activated or inhibited amongs all those other pathways, that would be huge,” he said.
In the meantime, optogenetics is taking neuroscience by storm, allowing scientists to pursue questions they never could before.
“It’s pioneered neuroscience — just brought it to life,” Grueter said.