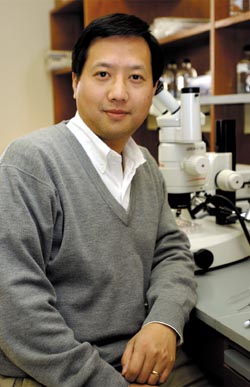
Tao P. Zhong, Ph.D.
(photo by Anne Rayner Pollo)
Blood vessel study uses zebrafish
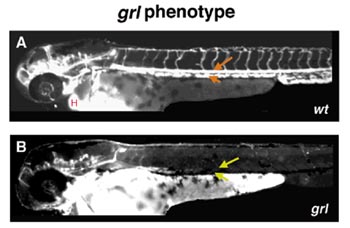
(Top) Circulation of green fluorescent beads in a normal zebrafish embryo.<br>(Bottom) Absensce of trunk circulation due to gridlock mutation, which caused malformation of vessels near the heart, as indicated by arrow. Green beads collect in yolk sac due to the blockage.
An obscure, torpedo-shaped fish that hails from India’s Ganges River is guiding new Vanderbilt investigator Tao P. Zhong, Ph.D., to a deeper understanding of blood vessel formation and its impact on cardiovascular disease.
Zhong, assistant professor of Medicine and Cell Biology, joined the faculty at Vanderbilt in September after a stint as a post-doctoral fellow at Harvard Medical School. There he worked with zebrafish embryos as a model for understanding a type of human blood vessel blockage seen in newborns called coarctation of the aorta. Though coarctation is a common occurrence routinely screened for by pediatricians, little is known of the cause.
“It’s a congenital cardiovascular disease,” Zhong said, “where a malformation, or blockage, occurs in the aorta during development. The child can survive with reconstruction of the area blood vessels—an adaptive mechanism takes place.”
A greater understanding of the cellular intricacies involved in formation of the blood vessels during embryonic development could lead to therapeutic intervention for this and other cardiovascular diseases, such as atherosclerosis and stroke.
Zhong and his colleagues published their work in the November issue of the journal Nature, where they described the crucial role of a gene— dubbed gridlock— that influences the fate of precursor cells destined to become blood vessels. A mutation in the gene had previously been shown to disrupt formation of major blood vessels in zebrafish embryos, causing a halt to circulatory “traffic.” Over the last several years, Zhong has used the animal model to methodically home in on how the protein encoded by gridlock is involved in blood vessel genesis.
Several features of the zebrafish, now a common denizen of hone aquariums, make it an ideal organism for developmental biologists like Zhong to use in studying how organs are formed during embryogenesis. The embryos are clear and develop outside of the mother’s body, allowing scientists to watch a zebrafish embryo grow into a newly formed fish under a microscope. This transparency makes observation of anatomical defects caused by genetic mutation easier. Moreover, zebrafish are particularly useful for studies of cardiovascular defects since survival of the embryos is not dependent on circulating blood flow.
At 24 hours of development, the beating zebrafish heart is clearly visible. The entire circulatory system can be visualized by injection of fluorescent microbeads, which leave a glowing green path from head to tail as the heart sends them coursing through the embryo.
Zhong and his colleagues used this microangiogram technique to study the effects of mutation in the gridlock gene in zebrafish. In the embryos with the mutation, they could see a collapse at the juncture of the two arterial vessels that come together to form the aorta. Subsequent injection of the microbeads demonstrated that the normal circulatory pathway had been blocked: no green fluorescence appeared in the trunk of the organism beyond that point.
“The interesting thing is, we found that this morphological defect was utterly restricted to arterial vessels,” Zhong said. “The adjacent venous branches were not affected.”
It had been generally thought that the artery and vein were made up of the same type of cell—the endothelial cell—and that their differences were simply a matter of structural alterations based on physiological influences. Arteries, for instance, which carry oxygenated blood away from the heart, have thicker walls than veins, and they have a stronger sheer force to tolerate the blood pressure created by the pumping heart.
“This was the first molecular evidence that defined the endothelial cells in arteries as being different from the endothelial cells in veins in the early stages of development,” Zhong said. “And we suspected that the gene was also expressed in the endothelial precursor cells.”
Since their ultimate goal is to intervene in the development of the vascular system before a malformation occurs, Zhong and his colleagues wanted to define the very early cellular events that signal endothelial precursor cells to become either an artery or a vein. They found that the gridlock protein was similar to other regulatory proteins that play key roles in cell-fate decisions during development, so they set about to see whether it, too, had a say in cell fate.
The researchers changed the building blocks—or nucleotides—of different genes and saw specific inhibition of aorta formation. One of these genes, called notch, encodes a signaling protein known to be responsible for cell-fate determination in other organisms, including humans. Through a series of experiments, they discovered that the two genes, notch and gridlock, interact in a single pathway that determines whether precursor cells are destined to become arteries or veins.
Specifically, they found that notch gene activation signaled the expression of gridlock, which drove the precursor cells toward developing into arterial vessels. Conversely, notch suppression reduced gridlock expression and disrupted the formation of the aorta.
Of course, questions remain. How, for instance, do the precursor cells know where to migrate, and how do they recognize and join other similarly fated cells? Zhong continues to explore the complex signaling pathway in the zebrafish model, and to think about how these findings might translate to human development and disease.
The gridlock gene, Zhong discovered, is present in every other organism studied, from the fruit fly to mouse to human. And the protein encoded by the gridlock gene is very similar—86 percent of the structure is identical—between zebrafish and humans. So understanding the zebrafish protein should give insight into the function of the human protein.
“We think it’s very possible that the mutation in the zebrafish gridlock gene might be similar to what happens in coarctation in humans,” Zhong said. “In collaborating with Dr. Doug Vaughan, chief of Cardiovascular Medicine, we are in the process of understanding the role of the human gridlock gene in this and other congenital cardiovascular diseases.”
Discovery of the pathway linking notch and gridlock function has opened a number of other research avenues for Zhong, many revolving around the question of why vascular diseases such as atherosclerosis and stroke occur only in arteries, not in veins.
Zhong looks forward to collaborating with the two other Vanderbilt scientists who use zebrafish in their studies, Bruce H. Appel, Ph.D., and Liliana Solnica-Krezel, Ph.D., both faculty members in the department of Biological Sciences.
“I’m very excited about being at Vanderbilt because the leadership is so supportive of the zebrafish model system,” Zhong said. “The support will allow us to explore not only the cardiovascular system, but also other organs, such as the kidney, pancreas, and liver. I’m really pleased with the research environment I’ve found here.”