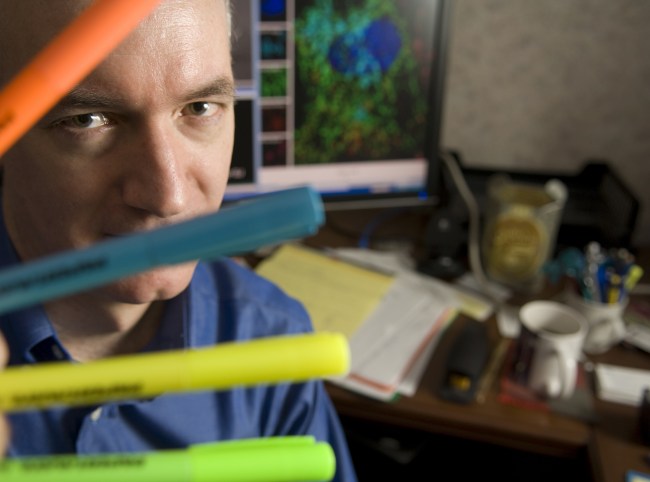
David Piston, Ph.D., and colleagues are studying fluorescent proteins that have the ability to shift colors. (photo by Susan Urmy)
Color-shifting fluorescent proteins highlight biology
A group of fluorescent proteins — useful probes for watching biology in action — have some newly discovered tricks.
They can shift from one fluorescent color to another, a process known as photoconversion, Vanderbilt researchers report in the May issue of Nature Methods.
This color-changing characteristic makes these proteins ideal for “highlighting” multiple cellular or subcellular populations to follow using imaging microscopy.
“With just two fluorescent proteins, we can label four different populations — such as secretory granules made at different times — and track their behavior,” said David Piston, Ph.D., professor of Molecular Physiology & Biophysics and the senior author of the current studies.
Piston and his colleagues will use the fluorescent tools to explore glucose-induced insulin secretion by the beta cells of the pancreas.
Fluorescent proteins — natural products of jellyfish, coral and other species — began to find their niche as biological imaging tools in the mid-1990s, when researchers discovered that they could genetically attach these proteins to cellular proteins and “see” them under the microscope. Green fluorescent protein (GFP), the first of the category, earned its discoverers and developers the 2008 Nobel Prize in Chemistry.
As investigators developed GFP-related proteins of different colors, they discovered how to “switch” some of these on and off, or convert them from one color to another.
Photoswitchable and photoconvertible fluorescent proteins — known as optical highlighters — allow direct and controlled highlighting of distinct molecular entities inside the cell, or of multiple cell types, and are particularly useful for investigating dynamic cellular processes.
Piston and colleagues examined the fluorescence characteristics of 12 fluorescent proteins that had not been previously shown to undergo photoconversion. They found that eight of the 12 exhibited photoconversion.
Most exciting, Piston said, the researchers discovered that two of the orange fluorescent proteins could be converted to emit a deep red light, with a “redder” wavelength than any other fluorescent protein.
Piston explained that red fluorescence is particularly desirable because biological tissues are most transparent to red light in the visible spectrum. (Consider the color of light that comes through when you hold your hand over a flashlight.)
The photoconverted red fluorescent protein is also noteworthy because the light required to convert it is in the visible range (not the ultraviolet range that is required for most photoconversions), and because it is very stable and works in acidic conditions, providing access to more places in the cell, Piston said.
“We're trying now to 'copy' the characteristics of that protein to make a bright stable deep red fluorescent protein from scratch,” he added.
The investigators also described three red fluorescent proteins that could be converted to a green state, which allows for many new experiments, but also raises concern about published studies that used these proteins but didn't take into account this potential for conversion.
“Some of these photoconverting characteristics are very useful for multiplexing — for labeling different populations and tracking them over time,” Piston said. “Others, though, are things you'd really want to avoid.”
He also noted that some of the photoconverting probes will be useful for tracking single molecules with super-resolution microscopy approaches.
Investigators interested in using photoconverting fluorescent proteins can find expertise and equipment in the Cell Imaging Shared Resource (http://cisrweb.mc.vanderbilt.edu/CISR/).
“We can do all of these things here at Vanderbilt,” Piston said.
The National Institutes of Health supported the research.