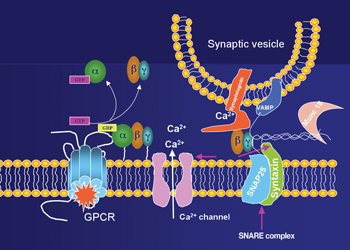
At left, activation of a G protein-coupled receptor releases the beta gamma subunit of an inhibitory G protein. The subunit blocks a vesicle protein, synaptotagmin, from binding to SNAP-25, part of the SNARE complex of proteins (right). This, in turn, prevents the complex from pulling the vesicle and the membrane together. The vesicle is thus unable to fuse to the membrane or spill its contents – neurotransmitter – into the synapse.
Discovery sheds light on neurotransmitter release mechanism
Researchers at three medical centers including Vanderbilt have discovered an important mechanism controlling the release of neurotransmitters and hormones.
The discovery, reported in the April and May issues of the journal Nature Neuroscience, could lead to new ways to treat pain, Parkinson's disease and perhaps even diabetes.
“This is a fundamental discovery … a very beautiful mechanism,” said one of the lead researchers, Heidi Hamm, Ph.D., Earl W. Sutherland Professor of Pharmacology and chair of the Department at Vanderbilt University Medical Center.
Neurotransmitters are chemical messengers that transmit electrical signals across the gap between nerve cells called the synapse. They are stored in tiny sacs called vesicles.
The electrical impulses, called action potentials, cause the vesicles to fuse with the nerve cell membrane and spill their contents into the synapse. The neurotransmitters then activate receptors on the other side of the synapse, essentially allowing the action potential to “jump the gap” to the next nerve cell.
In the brain, too little or too much neurotransmitter can contribute to disorders such as Parkinson's disease, depression and schizophrenia. These disorders are treated with drugs that mimic the action of deficient neurotransmitters or block overabundant ones from activating their receptors.
Nature, too, modulates the supply of neurotransmitter through G protein-coupled receptors. These receptors are named for their ability to activate G proteins — actually, to split them into two active parts, an alpha subunit and a beta-gamma subunit.
Hamm, who recently was named president-elect of the American Society for Biochemistry and Molecular Biology, helped solve the structure of the alpha subunit in 1993 with the late Paul B. Sigler, M.D., Ph.D., and his colleagues at Yale.
Four years ago, Hamm and colleagues including Simon Alford, Ph.D., of the University of Illinois at Chicago, and Thomas F. J. Martin, Ph.D., of the University of Wisconsin-Madison, reported that the beta-gamma subunit of an inhibitory G protein blocked neurotransmitter release from large, easily studied nerve cells of the lamprey eel.
But they didn't know the mechanism — until now.
In an elegant series of experiments, the researchers showed that the beta-gamma subunit prevents vesicles from fusing with the membrane of the nerve cell.
It does this by preventing another protein from binding to the cell's “fusion machinery,” a complex of proteins called SNARE that links the vesicle to the membrane. In particular, the beta-gamma subunit prevents synaptotagmin, a vesicle protein, from binding to the SNAP-25 protein in the SNARE complex.
The researchers identified the site of the subunit's action by injecting tiny amounts of a form of the paralyzing botulinum toxin that chopped off the end of the SNAP-25 protein. When that happened, the subunit could not block fusion, indicating that the chopped-off portion was its binding site.
This sounds complicated, and it is. But inhibiting vesicle fusion may be a major way the body regulates the release of a whole array of chemical messengers. Not only did the researchers confirm this mechanism in the nerve cells of a lamprey, but they also found that the subunit prevented secretion of norepinephrine, a hormone with similar effects to adrenaline, in a culture of rat adrenal tumor cells.
“We think it's going to have a general inhibitory effect,” Hamm said, perhaps even controlling release of insulin from beta cells in the pancreas.
Extrapolating findings in the lamprey and adrenal tumor cell systems to the human must be made with caution, University of Washington neuroscientist Jane Sullivan, Ph.D., wrote in a commentary on the research in the current issue of Nature Neuroscience.
Now, however, scientists can start to search for small molecules that may enable them to “turn up” or “turn down” the inhibition of neurotransmitter release at specific nerve cells in the brain, for example.
One possible target: endorphins, which bind inhibitory G protein-coupled receptors to dampen pain signals. Drugs that “turned up” the pain-blocking ability of endorphins could provide an alternative to the use of habit-forming narcotics such as morphine, codeine and hydrocodone.
Drugs that “turned down” the inhibition of dopamine release could be used to treat Parkinson's disease, which is characterized by a dwindling supply of the neurotransmitter. In this case, blocking the inhibitory subunit would effectively increase the supply of dopamine in the synapse.
“That would be a really powerful drug,” Hamm said.