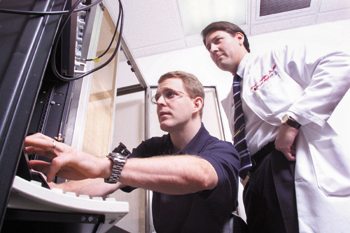
Graduate student Chris Lossin and Dr. Al George use electrophysiological techniques to study the consequences of genetic mutations that underlie inherited epilepsy. (photo by Anne Rayner Pollo)
Genetic reasons for ‘storms inside brain’ examined
They’re like lightning storms inside the brain. Epileptic seizures blow in suddenly, their rapid and rhythmic electrical bursts disrupting normal brain activity.
At the cellular and molecular level, these electrical storms are not completely understood, said Dr. Alfred L. George Jr., Grant W. Liddle Professor of Medicine and director of the division of Genetic Medicine. George and colleagues report last week in the journal Neuron how genetic mutations associated with an inherited form of epilepsy can perturb the electrical properties of brain cells. Their findings support a plausible mechanism for epilepsy and suggest novel tools for drug testing and development.
More than 2 million Americans have epilepsy, a disorder with multiple causes ranging from abnormal brain development to brain damage from illness or injury to genetic mutations. “The newest breakthrough in epilepsy research,” George said, “has been the identification of genes responsible for familial forms of epilepsy.”
Inherited epilepsy syndromes account for about 5 percent of all epilepsies, George said, though the exact number is unclear. By studying the defects that cause inherited epilepsy, George and others hope to learn about other, more common forms of the disorder. “There will be shared cellular and molecular mechanisms in all the epilepsies,” he said.
Of the identified genetic mutations associated with inherited epilepsies, many are in genes that encode ion channels — donut-like pores that let electric current, in the form of charged molecules called ions, flow into or out of the cell. Since these channels regulate the electricity that powers the brain, it makes sense that mutations affecting the channels lead to disturbances of brain function.
In 1998, a collaboration between George and colleagues in Australia identified the first sodium channel gene mutation associated with inherited epilepsy. Others subsequently identified additional sodium channel mutations linked to inherited epilepsy syndromes.
The current study focuses on three sodium channel mutations that are associated with a syndrome called generalized epilepsy with febrile seizures plus (GEFS+). Seizures associated with fever, febrile seizures, are the most frequent type of seizures in children between the ages of 6 months and 6 years. In families with GEFS+, seizures begin in association with febrile illness, but then persist beyond the age of 6 and/or occur in the absence of fever.
The investigators sought to understand — at the level of individual sodium channel molecules — the functional consequences of mutations that cause GEFS+. They isolated the human SCN1A sodium channel gene and introduced the gene, with or without epilepsy-associated mutations, into human cells in the laboratory.
The study is the first to examine the authentic human SCN1A sodium channel in a human cell, with other proteins called accessory subunits that are required for its function. And the channel protein in this environment looked like the real thing. “We were able to reconstitute sodium channel function in the laboratory in a way that is very similar to the natural neuronal cell in a human being,” George said.
With this system, the investigators examined sodium channels bearing one of three epilepsy-associated mutations. In each case, they found that the functional properties of the mutant channels were very similar to those of a normal channel, but that the mutant sodium channels failed to close completely, George said.
Sodium channels can be thought of as gates with spring-controlled hinges, George said. When the gates open, sodium rushes into the cell and enables the cell to fire an electrical discharge called an action potential, which drives brain activity. Shortly after they open, though, the sodium gates automatically swing closed — a process called inactivation. They remain closed for a period of time to protect the cell from a repeat action potential.
“The inactivation process is a way of safeguarding a cell from having repetitive firing,” George said.
In the case of the mutant sodium channels, the inactivation process is defective. The gates don’t swing closed. “It’s as if the hinges are rusty, or the gates hit a rock and can’t close completely,” George said. What this does, he explained, is allow sodium to continue to enter the cell, leading to a state of hyperexcitability that makes repetitive firing — a hallmark of seizure activity — more likely.
“This minor defect is sufficient to render the cell susceptible to repeat firing,” George said. “It is a plausible mechanism for epilepsy at the cellular and molecular level.”
George is excited about the possibility of using the cellular system as a drug-screening tool. A large number of anticonvulsant drugs used for treating epilepsy target sodium channels, he said. They are, in essence, “locks on the gate.” An ideal drug would eliminate only the abnormal closing properties of the sodium channels, not their initial openings that are important to normal brain function.
“We now have a physiologic event that we can target with a drug and test in the laboratory to identify drugs that are best at suppressing the abnormal non-inactivating current while leaving the normal current alone,” George said.
Such drugs might be important not only for treating epilepsy, but perhaps also for preventing it from developing in the first place. One theory holds that a sodium channel defect makes an individual more susceptible to having seizures, and that the seizures themselves then cause neuronal damage and create a focal point for future seizures.
“Preventing the damage could be an important issue, and perhaps someone known to have a sodium channel mutation like these should be on a prophylactic anticonvulsant — the right anticonvulsant,” George said.
Graduate student Christoph Lossin is the primary author of the Neuron paper. Other participants in the research include Dao W. Wang, Thomas H. Rhodes, and Carlos G. Vanoye. The work was supported by the National Institutes of Health and the Epilepsy Foundation.