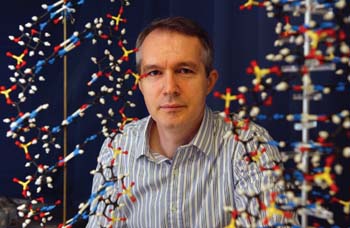
Research by Vanderbilt biochemist Martin Egli, Ph.D., is providing clues to the origin of DNA.
Photo by Dana Johnson
Investigator seeks to uncover roots of DNA’s ‘sweet’ secret
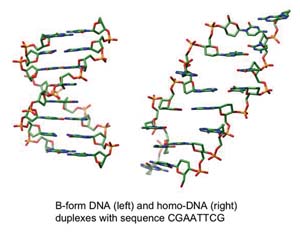
This graphic illustrates the differences between “normal” DNA, left, and homo-DNA.
DNA's simple and elegant structure — the “twisted ladder,” with sugar-phosphate chains making up the “rails” and oxygen- and nitrogen-containing chemical “rungs” tenuously uniting the two halves — seems to be the work of an accomplished sculptor.
Yet the graceful, sinuous profile of the DNA double helix is the result of random chemical reactions in a simmering, primordial stew.
Just how nature arrived at this molecule and its sister molecule, RNA, remains one of the greatest — and potentially unsolvable — scientific mysteries.
But Vanderbilt biochemist Martin Egli, Ph.D., isn't content to simply study these molecules as they are. He wants to know why they are the way they are.
“These molecules are the result of evolution,” said Egli, professor of Biochemistry. “Somehow they have been shaped and optimized for a particular purpose.”
“For a chemist, it makes sense to analyze the origin of these molecules.”
One particular curiosity: how did DNA and RNA come to incorporate five-carbon sugars into their “backbone” when six-carbon sugars, like glucose, may have been more common? Egli has been searching for the answer to that question for the past 13 years.
Recently, Egli and colleagues solved a structure that divulges DNA's “sweet” secret. In a recent issue of the Journal of the American Chemical Society, Egli and colleagues report the X-ray crystal structure of homo-DNA, an artificial analog of DNA in which the usual five-carbon sugar has been replaced with a six-carbon sugar.
By exchanging the sugars that make up the DNA backbone, researchers can make and test plausible “alternatives” to DNA — alternatives that nature may have tried out before arriving at the final structure. These alternative structures can then reveal why DNA's genetic system is more favorable than the other possible forms.
“If you can change the molecules chemically or functionally, you can see what is so particular about them, why are they optimal, why are they better than others,” he said.
Although homo-DNA was first synthesized in 1992, a detailed picture of the molecule's structure had been lacking. Egli's high resolution structure is now able to provide answers to some of the lingering questions about why DNA is made the way it is.
While the homo-DNA structure shows a number of similarities with DNA, it is much more stable than DNA. However, it has a more haphazard appearance than normal DNA, looking more like a “slowly writhing ribbon” than the tightly twisted ladder of DNA.
“The reason that DNA was 'picked' is not because it's thermodynamically extremely stable,” Egli said. “There are others — including homo-DNA — that are actually superior in that regard.”
Egli's structure also shows that homo-DNA has more flexibility in how the bases (rungs of the ladder) bind. The bases in normal DNA adhere to a somewhat strict binding scheme — guanine (G) binds with cytosine (C) and adenine (A) binds with thymine (T). In this “Watson-Crick” base pairing, the G:C bonds are much stronger than A:T or any other bonds.
“In homo-DNA, the Watson-Crick base pairing rules are changed,” Egli said. “For example, G:C is similar to G:G or A:A, so you have a much more versatile pairing system in homo-DNA. Therefore, the nature of the sugar in the backbone affects the pairing rules.”
But despite homo-DNA's apparent versatility in base pairing and its thermodynamic stability, other features of the molecule's architecture probably preclude it from being a viable genetic system
For example, it cannot pair with other nucleic acids — unlike DNA and RNA which can and must pair with each other. Also the steep angle, or inclination, between the sugar backbone and the bases of homo-DNA requires that the pairing strands align strictly in an antiparallel fashion — unlike DNA which can adopt a parallel orientation. Finally, the irregular spaces between the “rungs” prevent homo-DNA from taking on the uniform structure DNA uses to store genetic information.
The findings suggest that fully hydroxylated six-carbon sugars probably would not have produced a stable base-pairing system capable of carrying genetic information as efficiently as DNA.
“The structure now provides insight of a chemical nature that (the six-carbon sugar) is just too 'bulky.' It has too many atoms,” Egli said.
The new insights provided by this structure lie at the heart of the most fundamental of scientific inquiries — the origin of life on Earth. If the pieces of DNA and RNA hadn't come together just so, life as we know it would not exist.
Although Egli's structure has ruled out six-carbon sugars as viable alternatives for the sugar backbone of DNA, the existence of a plethora of sugars — as well as alternative bases — make for an almost endless number of possibilities from which nature selected the winning DNA combination.
“Homo-DNA is just one alternative system. There are hundreds of sugars, as many as you can think of. It will be almost impossible to look at all of them,” Egli said.
“But the big red herring of this work could be that nature never went through these other sugars. Maybe it just hit on gold (these five-carbon sugars) very early and took off from there.”
The research was funded by the National Institutes of Health and the American Chemical Society Petroleum Research Fund.