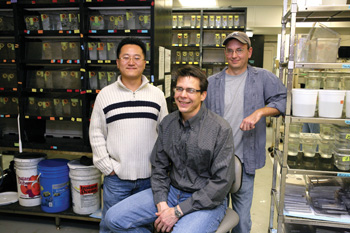
Bruce Appel, Ph.D., center, with research fellow Andrew Latimer, right, and graduate student Jimann Shin.
Photo by Daniel Dubois
Nerve insulation process highly dynamic
Much like the electrical wiring in your house, the nerves in your body need to be completely covered by a layer of insulation to work properly.
Instead of red, white or black plastic, however, the wiring in the nervous system is protected by layers of an insulating protein called myelin. These layers increase the speed that nerve impulses travel throughout the brain and the body. The critical role they play is dramatically illustrated by the symptoms of multiple sclerosis, which is caused by lesions that destroy myelin. These include blindness, muscle weakness and paralysis, loss of coordination, stuttering, pain and burning sensations, impotence, memory loss, depression and dementia.
The formation of myelin sheaths during development requires a complex choreography generally considered to be one of nature's most spectacular examples of the interaction between different kinds of cells. Now, a group of Vanderbilt researchers has successfully produced movies that provide the first direct view of the initial stage of this process. That's the period when the cells that ultimately produce the myelin sheathing spread throughout the developing nervous system. The results were published online recently in the journal Nature Neuroscience and could aid in the design of new therapies to promote the repair of this protective layer following disease or injury.
“We discovered that this process is far more dynamic than anyone had dreamed,” says Bruce Appel, Ph.D., the associate professor of Biological Sciences and Kennedy Center investigator who headed up the study.
In the central nervous system, the myelin membranes are produced by cells called oligodendrocytes. These cells must be distributed uniformly along axons — the long, wire-like extensions from neurons that carry nerve impulses — so that the membranes, which wrap the nerve fibers like millions of microscopic pieces of electrician's tape, can cover the axons completely and uniformly. The wrapping process takes place near the end of fetal development and actually continues for some time after birth.
In order to study this process, Appel and his research group — graduate students Brandon Kirby and Jimann Shin along with post doctoral fellows Norio Takada and Andrew Latimer — created a transgenic zebrafish which incorporates fluorescent proteins in the cells involved in myelination.
The oligodendrocytes that produce the myelin membranes arise from mobile, dividing cells called “oligodentrocyte progenitor cells” or OPCs. OPCs are made in special locations in the brain and spinal cord. These cells seek out axons and spread out along them.
Then, at a certain point, a fraction of the OPCs transform themselves into oligodentrocytes and begin wrapping axons. Each cell can wrap portions of several different axons and each axon is wrapped by a large number of oligodentrocytes.
Previous studies of OPCs grown in tissue culture had seen that they could generate small, tentacle-like pseudopods, called filopodia, but no one knew what their purpose might be. So, when the researchers began viewing their movies, they were excited to observe that the cells were continually sending out filopodia in different directions. They observed that when one of these tiny tentacles touches a neighboring OPC, the cells react by moving in the opposite direction. This caused a surprising amount of movement as the OPCs repeatedly readjusted their positions.
“This could serve as a surveillance mechanism that allows the OPCs to determine the presence or absence of nearby cells of the same type,” says Appel, “and could explain how they distribute themselves along the axons.”
The researchers used the same system to see how the OPCs respond to injuries and conditions like multiple sclerosis. They did so by using a laser to destroy the OPCs along a short length of the embryo's spine a day before the axon-wrapping stage begins. They found that the OPCs in the vicinity of the gap start dividing to produce additional cells that move into the gap.
After a day, the number of OPCs in the gap had grown to 50 percent of normal and after four days it had risen to 70 percent.
“Now that we have a better understanding of OPC and oligodendrocyte behaviors, we are in a much better position to identify and study the genes that are necessary for myelination,” says Appel, “and having these genes in hand should aid in the design of drugs to promote remyelination following disease or injury.”