VUMC researchers study protein that exhibits self-control
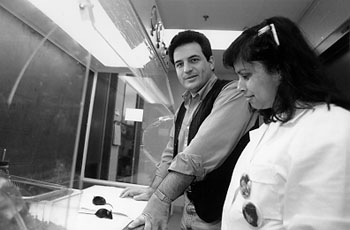
Ronald Emeson, Ph.D., here in his lab with Minati Singh, is investigating a cellular editor protein that actually prevents itself from making too many changes. (photo by Donna Jones Bailey)
Like newspapers and magazines, the body's cells have "editors" that tweak and refine story content.
In cells, these "stories" are instructions for making proteins, and an overzealous editor can sometimes do more harm than good. Recently, Vanderbilt University Medical Center scientists discovered that a cellular editor protein actually prevents itself from making too many changes.
The editor changes single nucleotide "letters" in RNA, the copy of DNA that gets decoded to make proteins. Malfunctions in the process of RNA editing may underlie seizures and mental illnesses like schizophrenia and depression.
"RNA editing is a relatively recent scientific discovery," said Ronald B. Emeson, Ph.D., Joel G. Hardman Professor of Pharmacology and associate professor of Molecular Physiology and Biophysics.
A description of RNA editing in 1991 so fascinated Emeson that he decided to change the research directions of his laboratory to work on this emerging field.
"The Human Genome Project assumes that once we know the sequence of the entire genome, we'll be able to predict every protein sequence. With the discovery of RNA editing, that's not going to be true by a long shot," Emeson said.
"RNA editing events change individual nucleotides within the RNA, changing as little as a single amino acid in the final protein and dramatically altering the biological activity of resultant proteins."
For example, the editing of RNAs encoding a particular glutamate receptor subunit is crucial to the normal function of the receptor. In response to the excitatory neurotransmitter glutamate, sodium and potassium ions pass through the edited receptor channel. If the receptor is not edited, calcium ions can also pass through, with lethal consequences.
"Peter Seeburg's laboratory at the University of Heidelberg generated genetically modified mice that couldn't edit this receptor subunit, and the animals died from seizures shortly after birth," Emeson said. "That's currently the best example that editing is absolutely critical for normal brain function."
In collaboration with Elaine Sanders-Bush, Ph.D., professor of Pharmacology and Psychiatry, Emeson discovered that another neurotransmitter receptor, the serotonin-2C receptor, is edited. Several editing events change up to three amino acids and affect how the serotonin-2C receptor signals.
"Various regions of the brain express different edited serotonin-2C isoforms, suggesting that these receptor isoforms have different functions. Because the serotonin-2C receptor is involved in a number of neuropsychiatric disorders – schizophrenia, depression – it's possible that alterations in editing underlie some of these pathological effects," Emeson said.
Understanding RNA editing may shed light on these neurological disorders and point the way to new treatments, Emeson said. As part of efforts to explore this process, he has focused on the editor protein ADAR2 and reported new findings in the May 6 issue of Nature.
ADAR2, short for adenosine deaminase that acts on RNA, is one of two related families of RNA editing enzymes that change the nucleotide adenosine (A) into inosine (I).
While characterizing the RNAs that encode rat ADAR2, Susan M. Rueter, Ph.D., a former graduate student at VUMC, identified four different forms. She determined that these RNAs are produced from the single ADAR2 gene by alternative splicing.
Splicing is a common mechanism used to remove the gibberish in between meaningful "letters" in genes. It can be thought of as cutting out sections of the RNA chain and rejoining the two ends. Because many genes can be alternatively spliced (cutting out different lengths of the chain), finding four forms of ADAR2 was not particularly remarkable, Emeson said.
What was surprising was that one of the splicing events could muck up the ADAR2 protein. This splicing event near the beginning of the ADAR2 RNA either cuts out or leaves in 47 nucleotide links in the chain. RNA that includes the 47 nucleotides encodes a short ADAR2 protein with no editing activity.
"The thing that became really interesting and perplexing is that 80 percent of the ADAR2 RNA in the brain includes the 47 nucleotides that shut off the protein," Emeson said. "Why would the brain go to the trouble of making an RNA, and then alternatively splice it so that it doesn't encode anything useful?"
The story became even more complicated when Rueter determined that the alternative splicing of ADAR2 was dependent upon the ability of ADAR2 to edit its own RNA, creating a new "splice acceptor" – the place in the chain that is cut and rejoined.
"These data describe a negative feedback mechanism by which ADAR2 controls its own level of expression," Emeson said. "Too much ADAR2 in the cell can edit RNAs that are not normally modified, producing deleterious consequences for the organism. So when ADAR2 levels get too high, it edits its own RNA to shut down its expression," Emeson said.
The group is currently studying what happens to cells and animals when ADAR2 can't shut itself off. In addition to Rueter, now a postdoctoral fellow at the University of Pennsylvania, Renee Dawson, a graduate student in Emeson's lab, contributed to the studies. The work was supported by the NIH.