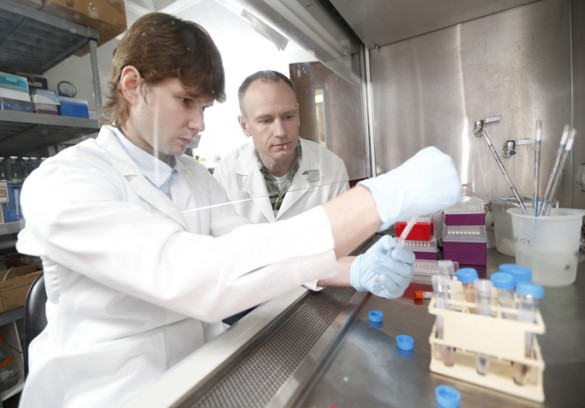
Vanderbilt University investigators have developed a new strategy for identifying the “bits” of a pathogen that spark a protective immune response.
Their research, reported in the May issue of the Journal of Clinical Investigation, provides a framework for the rational design of vaccines that engage immune system T cells.
T cells and B cells comprise the “adaptive immune system,” which recognizes and responds to pathogens. B cells generate antibodies that bind pathogens directly, marking them for destruction. T cells recognize pieces of a pathogen’s proteins that have been chopped up and “displayed” on the surface of antigen-presenting cells. Some T cells kill infected cells; others help ramp up the overall immune response.
“The age-old view to vaccine design has been to generate antibody responses,” said Sebastian Joyce, Ph.D., professor of Pathology, Microbiology and Immunology, and senior author of the new study. “But we know from years of research that T lymphocytes play a critical role, especially in viral clearance and immunity to viruses.”
Because antibodies bind to a pathogen’s surface proteins, these proteins — or the entire pathogen — are used for antibody-generating vaccines. It’s not clear, however, how to design a vaccine that will generate a protective T cell response, Joyce said.
“Huge pathogens like vaccinia virus or the parasite that causes malaria can generate hundreds of thousands of different T cell epitopes (the pieces of pathogen that are displayed by antigen-presenting cells),” said first author Pavlo Gilchuk, Ph.D., a postdoctoral fellow in Joyce’s group. “For rational vaccine design, we need to know which of these epitopes generate a protective immune response.”
Although bioinformatics approaches can be used to predict potential epitopes, it is too cumbersome to experimentally test each of the predicted epitopes for a protective response, Gilchuk said.
To narrow the search for protective epitopes, the investigators opted to “let the cell tell us what it can naturally do,” Joyce said.
The researchers infected antigen-presenting cells with vaccinia virus (a relative of smallpox) and allowed the cells to process the virus and produce epitopes bound to MHC (major histocompatibility complex) proteins. Then, with Andrew Link, Ph.D., and his team at Vanderbilt, they used mass spectrometry with direct sequencing to identify the naturally processed epitopes.
Next, they used functional assays — with cells from human volunteers who had been vaccinated for smallpox and in a mouse model — to determine which naturally processed epitopes were recognized by T cells. Then they confirmed that a subset of the recognized epitopes — injected as a vaccine — were able to protect mice against a lethal vaccinia virus challenge.
“Our study is a technical advance; it’s the first proof-of-principle for how to search for protective T cell epitopes for complex pathogens,” Gilchuk said. “This can have a great impact on developing protective T cell vaccination strategies for stubborn infectious diseases for which we don’t have any vaccines yet, like malaria and tuberculosis.”
Developing T cell vaccines is further complicated by the variability in human MHC molecules, the researchers pointed out. A global vaccine would need to include multiple epitopes that are presented by different types of MHC molecules, they said.
The new strategy should be generally applicable to rational vaccine design, Joyce said.
“The goal is to define the protective epitopes and to build a vaccine based on those epitopes. We think we’re on the right track.”
The studies “took a village,” Joyce said. In addition to members of Joyce’s and Link’s research teams, other Vanderbilt contributors to the research included James Crowe Jr., M.D., John Williams, M.D., Kelli Boyd, Ph.D., DVM, and the Flow Cytometry Shared Resource. Ton Schumacher, Ph.D., the Netherlands Cancer Institute, Soran Buus, Ph.D., University of Copenhagen, William Hildebrand, Ph.D., University of Oklahoma, and Alessandro Sette, Ph.D., La Jolla Institute of Allergy and Immunology, also made critical contributions to the studies.
The research was supported by the National Institutes of Health through research grants (HL054977, AI042284, AI061721) and contracts (AI040079, AI0400024, AI025462).