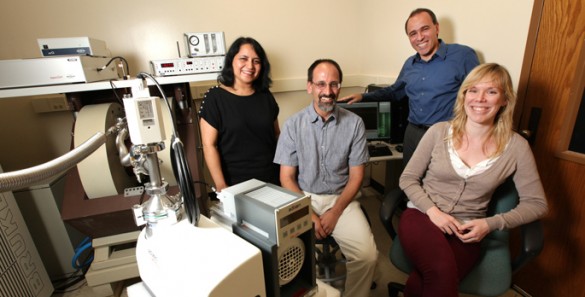
Vanderbilt University researchers and their Belgian colleagues have discovered the mechanism behind a multidrug transporter. Their findings, posted this week by Nature Chemical Biology, could lead to new treatments for multidrug resistant bacterial infections.
Infections that do not respond to typical antimicrobials, called multidrug resistant infections, are very difficult to treat. To fight these infections more effectively, researchers need to know more about how bacteria become resistant to so many different types of drugs.
One possible mechanism of resistance is via proteins acting like tiny pumps, called transporters, located in the bacterial cell membrane. These transporters are able to pump antibacterial drugs out of the cell, rendering the drugs useless.
While most transporters are specific to one type of chemical, multidrug transporters are able to pump out many different types of drugs. Until now, researchers did not know how transporters do this.
The paper’s senior authors were Hassane Mchaourab, Ph.D., professor of Molecular Physiology and Biophysics at Vanderbilt, and Cédric Govaerts, Ph.D., associate professor of the Fonds de la Recherche Scientifique in Brussels, Belgium.
The experiments were conducted by first authors Matthieu Masureel and Chloé Martens, Ph.D., students at the Université Libre de Bruxelles in Belgium, and Richard A. Stein, Ph.D., research instructor in Molecular Physiology & Biophysics at Vanderbilt.
The researchers focused on a specific type of transporter called LmrP from the gram positive bacterium, Lactococcus lactis. This transporter is able to pump out a large number of drugs including antibiotics and anticancer drugs. It is activated by an electro-chemical proton potential across the cell membrane.
For the transporter to work, it needs to bind the drug from the cytoplasm and release it to the extracellular medium.
Using a technique for measuring protein dynamics called Double Electron-Electron Resonance, or DEER, the team followed how LmrP changes its shape or conformation to accomplish this task and figured out how the proton energy source drives the change in shape.
In the model proposed by the researchers, LmrP has two openings: one opens to the outside of the cell, and another to the inside. At rest, both openings are closed. But when a molecule such as an antibiotic binds, LmrP opens to the outside of the cell.
Since there are more protons outside the cell than inside, they are drawn into the transporter. They then compete for space with the antibiotic causing the antibiotic to be expelled into the extracellular space.
The proton hops along a relay of conserved acidic amino acids until it reaches the intracellular side. This “proton transfer” triggers the conformational change that allows the transporter to close on the extracellular side.
The proton is then released to the inside of the cell resulting in the closure of the inside opening so that another cycle can begin.
According to conventional wisdom, antibiotic binding causes the transporter to change shape. “But in fact we have shown that the main driver of the conformational change is the proton transfer,” Martens said.
By understanding proton-coupled drug resistance in more detail, researchers may be able to find a way to block the transporter, providing a strategy to prevent multidrug resistance.
This work was supported by National Institutes of Health grant GM077659, and by a Belgian grant, Mandat d’Impusion Scientifique, from the Fonds National de la Recherche Scientifique.
— by Emily Mason